Circulating tumor cells et al.: towards a comprehensive liquid biopsy approach in breast cancer
Introduction
Over the course of the past decade, liquid biopsy has gained increased prominence in the management of patients with breast cancer (BC) (1). This minimally invasive approach for detecting circulating tumor biomarkers has proven invaluable in unraveling key information about tumor biology. Its validity extends to prognosis, prediction, and monitoring of treatment response in patients with BC. One of the noteworthy attributes of liquid biopsy is its ability to surmount the inherent limitations associated with traditional tissue biopsy. By circumventing challenges such as the inability to capture spatial tumor heterogeneity and the impracticability of serial sampling, liquid biopsy offers a real-time lens into the evolving landscape of tumors, providing a dynamic and comprehensive assessment of tumor evolution (2).
Even though nowadays the term liquid biopsy is predominantly associated with the detection of circulating tumor DNA (ctDNA), it was originally coined to describe the presence of circulating tumor cells (CTCs) (3). CTCs have been shown to play a pivotal role in the metastatic process, intravasating into the bloodstream and disseminating at distant sites (4). Many studies in patients with BC have focused on the detection of CTCs and CTCs detected using the Food and Drug Administration (FDA)-approved CellSearch® system have emerged as an independent and strong biomarker for survival in patients with early and metastatic BC (MBC) (5). Beyond the established prognostic role, also the predictive value of CTCs has been evaluated both based on the enumeration (6) and expression of surface biomarkers of CTCs, notably the human epidermal growth factor receptor 2 (HER2) (7).
Despite advancements in the CTC field, the main challenge lies in their low abundance, especially in the early setting. In fact, CTCs are elevated in up to 50% of patients with MBC (8,9) (cutoff of 5 CTCs per 7.5 mL of blood) and 25% of early-stage BC (EBC; cutoff of 1 CTC per 7.5 mL of blood) (10,11). Moreover, although these cutoffs have been validated and used for many years, discrepancies between CTC number and outcomes for individual patients were frequently observed. Therefore, additional markers are needed to stratify patient prognosis more precisely. Recognizing this, the utility of combining various circulating biomarkers, including cell-free DNA (cfDNA), extracellular vesicles (EVs), cell-free RNAs (mRNAs, long noncoding RNAs, and microRNAs), and other non-malignant circulating cells, becomes evident. Moreover, tumors are complex, and CTCs defined by positivity for epithelial cellular adhesion molecule (EpCAM) and cytokeratins (CKs) represent only a fraction of circulating cells involved in disease dissemination.
The simultaneous evaluation of different liquid biopsy analytes can maximize the information derived from a single peripheral blood sample and provide a more accurate picture of the complexity of BC (Figure 1) (12). While the clinical significance of these circulating biomarkers has been largely assessed independently, understanding their potential complementarity could enhance prognosis definition thus enabling a more precise management of BC patients.
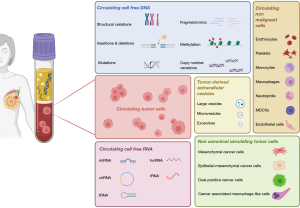
This article provides an overview of studies investigating the clinical value of concomitant analysis of CTCs and other circulating biomarkers. It delves into the opportunities offered by a comprehensive liquid biopsy approach in BC and the associated challenges.
CTCs and ctDNA
ctDNA constitutes a small fraction of the cfDNA, released into the bloodstream by cancer cells after cell death (13). The presence of ctDNA has been extensively studied, revealing its prognostic significance in both EBC and MBC (14). Furthermore, the analysis of ctDNA alterations enhances the identification of potentially actionable mutations, making it a valuable tool for guiding treatment selection.
CTC and ctDNA levels
Dawson et al. pioneered a proof-of-concept study, revealing ctDNA as an informative, specific, and highly sensitive biomarker in MBC (15). This study analyzed ctDNA and CTCs in 30 patients with MBC demonstrating that ctDNA outperformed CTCs in detection frequency, dynamic range, and correlation with tumor burden. Larger studies confirmed the prognostic significance of ctDNA detection, although in contrast to CTCs a cutoff of ctDNA correlated with worse prognosis has not been validated yet (16,17). Several studies have thus investigated the complementary prognostic value of ctDNA and CTC levels in MBC demonstrating that their combination is more informative than a single analyte for the prediction of patient outcomes (18-23). Nevertheless, the clinical validity of such a multimodal liquid biopsy approach has not been established yet. Shaw et al. reported a significant correlation between CTC count and cfDNA levels, establishing both as independent indicators for overall survival (OS) in MBC patients (24). Ye et al. evaluating 117 patients with MBC confirmed the individual impact of CTC and cfDNA on progression-free survival (PFS) (hazard ratio =2.58 and 2.05, respectively) and OS (hazard ratio =3.63 and 3.56, respectively), independently of clinical covariates and CTC/cfDNA levels, emphasizing the non-overlapping prognostic value of CTCs and cfDNA (25). Moreover, this analysis highlighted the joint effect of CTCs and cfDNA levels: patients with high levels of both markers had a >17-fold increased death risk (P<0.001) compared to those with low CTC and ctDNA levels. This joint effect was less prominent on PFS than OS suggesting that both CTC and cfDNA provide more values that are prognostic but not predictive of response to specific treatments. Additionally, longitudinal analysis from 22 patients revealed that responders exhibited lower CTC and cfDNA levels at first follow-up than non-responder patients and interestingly suggested that the inconsistency between CTC changes and treatment response in some patients could be largely explained by cfDNA level. In the prospective COMET study, an analysis of 198 HER2-negative MBC patients receiving first-line chemotherapy showed a moderate correlation between CTCs and ctDNA detection at baseline and four weeks of treatment and confirmed the validity of both blood biomarker levels as prognostic markers before and during therapy (26). Moreover, combining CTCs and ctDNA improved the prognostic accuracy of multivariate clinicopathological models, with the best models for PFS and OS incorporating ctDNA variant allele frequency (VAF) at baseline (as a continuous variable) and CTC count at four weeks (as a dichotomized variable). Remarkably, only 13% of patients had neither ctDNA nor CTCs detected at baseline, suggesting that combining both markers could enhance the number of patients assessable for marker detection and monitoring. Gerratana et al. evaluated the longitudinal evolution of CTCs and ctDNA and their impact on outcome prediction (27). They observed that while mutant allele frequency (MAF) in ctDNA appeared to follow treatment response versus progression, the number of CTCs increased only at the time of clinical progression. This suggests that ctDNA might be more suitable for real-time assessment of tumor burden and treatment effectiveness, while CTC counts could be more indicative of metastatic biology, offering an overall readout of the clinical aggressiveness of the disease.
CTCs and ctDNA complementarity has been assessed also in the early setting. Compared to CTCs, which are detectable in almost a quarter of patients with EBC (10,11), ctDNA can be identified in the majority of EBC patients thus representing a broadly applicable biomarker for disease monitoring (28-31). However, CTCs may provide additional information about the presence of minimal residual disease (MRD). In a secondary analysis of the BRE12-158 phase II clinical trial, the detection of ctDNA and CTCs in patients with early-stage TNBC after neoadjuvant chemotherapy was independently associated with disease recurrence (32). Notably, there was no significant association between CTC positivity (defined as ≥1 CTC detected) and ctDNA positivity, with a proportion of patients positive for only one marker. Thus, combining both markers significantly increased sensitivity for recurrence detection, from 79% with ctDNA alone and 62% with CTC alone to 90% when combined. The combined analysis demonstrated a stepwise reduction in distant disease-free survival (DDFS), with patients positive for both ctDNA and CTCs having a median DDFS of 32.5 months, compared to those positive for one marker or negative for both [median DDFS not reached for ctDNA−/CTCs−; hazard ratio =5.29; 95% confidence interval (CI): 1.50–18.62; P=0.009]. This association was stronger than the detection of ctDNA alone (hazard ratio =2.99), with similar trends observed for disease-free survival (DFS) and OS when comparing ctDNA+/CTCs+ to ctDNA−/CTCs−. Another recently published study offered insights into the role of comprehensive liquid biopsy analysis for the therapeutic management of EBC patients (33). Over a ten-year period, the study examined 13 EBC patients through multianalyte liquid biopsy approach, encompassing enumeration, phenotypic characterization, and molecular monitoring of CTCs along with corresponding ctDNA mutation and methylation profile. Among the ten patients negative for liquid biopsy biomarkers throughout the follow-up, no relapse was observed. In contrast, three patients who tested positive for at least one liquid biopsy biomarker experienced relapse during the follow-up period. The comprehensive approach used in this study demonstrated the potential to identify MRD four years before clinically detectable metastatic disease manifests. These findings suggest that beyond the established role of ctDNA in the detection of MRD in the circulation after surgery (34-36), the definition of a combined liquid biopsy-MRD might provide additional information and have a higher discriminatory capacity. This might lead to a broader definition of MRD through the combination of ctDNA (molecular residual disease) and CTCs (cellular residual disease) (37).
CTC and ctDNA alterations
Various studies have explored the association between CTC count and ctDNA alterations, aiming to uncover the biological mechanisms that underlie their presence. Utilizing the PredicinePLUS™ platform for ctDNA sequencing, a study revealed a significant association between ctDNA alterations in ESR1 and GATA3 and an increased number of CTCs (38). Furthermore, alterations in CDH1 were associated with a higher occurrence of CTC clusters, defined as aggregates of two or more CTCs. A report by Bortolini Silveira et al. demonstrated an association between specific ctDNA alterations and CTC counts: lysine (K)-specific methyltransferase 2 C gene (KMT2C/MLL3) variants were significantly associated with reduced CTC count, while the opposite trend was seen with GATA3 alterations (26). Although the latter association did not reach statistical significance (P=0.07), it supports the finding of Davis et al. (38) and may be related to the supposed role of GATA3 in the migration and dissemination of BC cells (39). Regarding KMT2C, its inactivation in a gastric model was shown to promote epithelial-to-mesenchymal transition (EMT) and the acquisition of stem cell-like phenotypes (40), potentially explaining the lower CTC detection rate using the EpCAM-based CellSearch® system. Notably, KMT2C is frequently mutated in hormone receptor (HR)-positive BC and associated with resistance to endocrine therapies (41).
Moving beyond the mere enumeration of CTCs, researchers have explored the simultaneous molecular characterization of both CTCs and ctDNA. Some studies reported an advantage in analyzing emerging resistance mutations in CTCs compared to cfDNA (42,43). Paolillo et al. analyzed single CTC DNA and matched ctDNA in patients with HR-positive MBC, confirming all ESR1 mutations in ctDNA within the endocrine receptor-positive (ER+) CTC population, establishing a mechanistic link between CTCs and ctDNA (44). A subsequent study comparing ESR1 mutations in BC patients demonstrated higher sensitivity in ctDNA compared to CTCs: at progression after endocrine therapy the ESR1 mutation detection rate based on ctDNA and CTCs was 42% and 8%, respectively (45). This suggests that analyzing a large number of CTCs may be necessary to identify the full range of mutations in MBC. Another study comparing PIK3CA hotspot mutations in CTCs and paired ctDNA reported higher concordance in the metastatic setting (66.6%) than in the early setting (48.2%) (46), emphasizing the importance of CTC levels.
Recent research revealed the complementary nature of cfDNA and CTCs in identifying mutations in MBC, with a 28% overlap of mutations between the two fractions, while the majority of variants were unique in either cfDNA or CTCs (47). Interestingly, ERBB2 variants were exclusively detected in CTCs, while BRCA1/2 variants were more frequently found in CTC than in cfDNA, and PIK3CA and ESR1 mutations were more common in cfDNA compared to CTCs. This may suggest that the choice between different analytes may depend on the specific clinical question. Moreover, a small percentage of patients (6%) showed no variants in either cfDNA or CTCs, highlighting the potential of multiparametric mutational analysis to optimize the identification of actionable targets, maximizing the benefit of targeted therapies.
Moreover, information residing in ctDNA that can be coupled with CTCs, extends beyond variants detection. Methylation of cfDNA (48), analysis of cfDNA fragments length (49), and end motifs (50,51) can provide additional layers of information. Studies comparing methylation profiles in matched cfDNA and CTCs revealed a high correlation in SOX17 promoter methylation in both early and MBC, associated with outcomes in the latter setting (52,53). Additionally, a high concordance (98.3%) in ESR1 methylation in cfDNA and CTCs was reported in BC patients, with ESR1 methylation in CTCs associated with a lack of response to everolimus-exemestane (54).
CTCs and EVs
EVs are membrane-enclosed structures of different size and origin released from cells, which play a crucial role in intercellular communication (55). Different subclasses of EVs play various roles in disease progression and metastatic processes. Small EVs, especially exosomes, have been the main focus of BC research. However, the complex and time-consuming techniques needed for their isolation, enumeration, and phenotype posed scalability issues for clinical use and alternative approaches are currently being investigated (56-58). Large tumor-derived EVs (tdEVs), though much less abundant than small EVs, offer potential advantages. With a diameter exceeding 1,000 nm, tdEVs can be isolated alongside CTCs using the CellSearch® system. Initially identified through the ACCEPT software in castration-resistant prostate cancer (CRPC) patients (59), tdEVs are defined as 1–12 µm particles coexpressing CK and EpCAM but lacking the leukocyte marker CD45 and DNA stain. Although, because of the isolation method, tdEVs have not been fully characterized, their size is consistent with large oncosomes. Notably, tdEVs were around twenty times more frequent than CTCs in these patients, significantly correlating with survival. Subsequent studies by Nanou et al. confirmed the high frequency and prognostic value of tdEVs in MBC (60). A normal range of tdEVs (<20 per 7.5 mL of blood) was established in healthy controls, while in MBC patients, a cutoff of ≥80 tdEVs led to a significant dichotomization of patients with <5 CTCs, indicating a 4.9-fold higher risk of death in those with unfavorable tdEV counts. This finding suggested the value of combining CTCs and tdEVs for a more accurate assessment of patient outcomes. An analysis of the SWOG S0500 clinical trial affirmed the independent and complementary prognostic significance of tdEVs hormone-insensitive MBC receiving first-line chemotherapy (61). In the overall population, the median OS significantly varied based on tdEV counts: 43.3, 29, and 17.1 months for low [0–19], intermediate [20–79], and high [≥80] tdEVs, respectively. Of note, among patients with 0–4 CTC (favorable prognosis, median OS =34.2 months), those with high/intermediate tdEVs had significantly worse OS (23.2 and 30.9 months, respectively) than patients with low tdEVs (42.5 months). This suggests that among patients considered to have a more indolent disease based on low CTCs, there is a higher-risk subpopulation that might be identified using tdEVs. Even in patients with ≥5 CTCs (poor prognosis), tdEVs provided additional prognostic insights, with a median OS of 26.5 and 16.0 months for patients with intermediate and high tdEVs, respectively. Intriguingly, four patients with high CTCs and low tdEVs reported an exceptionally long median OS of 83.7 months. Further research should be aimed at unraveling the composition and exact origin of these abundant subcellular objects to elucidate their biophysical properties and contribute to better understand the mechanisms underlying their prognostic significance.
Incorporating tdEVs into the management of patients with MBC could offer additional benefits. TdEVs, being more abundant than CTCs, might better reflect tumor heterogeneity and increase the number of patients’ samples evaluable for therapeutic targets, since only a small number of patients have undetectable tdEVs. Studies have reported the expression of tumor-associated antigens such as HER2 and programmed death-ligand 1 (PD-L1) on tdEVs. As for CTCs, these biomarkers can be identified and quantified on tdEVs using CellSearch®. Investigations from Terstappen and his group showed that HER2 staining of tdEVs outperformed CTC staining in predicting the HER2 status of BC: a threshold of ≥7% of HER2-positive tdEVs predicted tissue HER2 expression with 74% specificity and sensitivity (62). However, a limitation was the use of HER2 amplification status from primary tissue, often diagnosed years before CTC and tdEV analysis. Given HER2’s dynamic expression changes between primary and metastatic disease, further validation is essential to confirm concordance. Moreover, as compared to CTCs, the higher tdEV levels might make the latter a better indicator of tumor burden and possibly a more sensitive biomarker for treatment monitoring (63). Finally, the increased stability of EVs in the circulation may facilitate the evaluation of the mutational status, transcriptome, and proteome profile of the tumor. The characterization of tdEVs could unravel the presence of therapeutic targets and contribute to the comprehension of mechanisms that promote resistance and progression in BC. Previous studies identified androgen receptor (AR) or epidermal growth factor receptor (EGFR) mutations in CellSearch®-enriched blood samples of CRPC (64) and non-small cell lung cancer patients (65), respectively, that did not have any CellSearch®-defined CTCs. Though these gene mutations could also derive from CK- CTCs (66), it is possible that these are encapsulated within tdEVs, as supported by the presence of most tumor DNA in large CK+ tdEVs found in the plasma of prostate cancer patients (67).
Of note, these large tdEVs isolated with CTCs from the blood cell pellet represent only a small subset of probably <1% of the total EVs present in the blood before centrifugation. A larger fraction of smaller EVs, including exosomes and microvesicles, reside in the plasma fraction and their association with CTCs remains less explored. In 2018 a study compared messenger RNA (mRNA) profiles of matched CTCs and EVs (from plasma fraction) in patients with HR-positive/HER2-negative MBC for the first time (68). The analysis revealed only a 5% overlap in signals between CTCs and EVs, emphasizing significant differences in mRNA profiles and the complementary nature of these analytes. Interestingly, divergent clinical outcomes were observed for mTOR transcript overexpression in CTCs versus EVs. Moreover, EV levels from plasma fraction pre- and post-neoadjuvant therapy have been associated with outcomes in patients with EBC suggesting that EVs may represent a complementary marker for providing information on MRD status (69). In this study an inverse association between stem cell-like and/or resistant CTCs and EV concentrations after therapy was observed, hypothesizing that these cells may internalize EVs.
Beyond canonical CTCs
Most clinical studies on CTCs in BC have been conducted using the CellSearch® system, defining CTCs as nucleated cells expressing EpCAM and CK but lacking CD45. Despite the unquestionable value of such definition (9), emerging evidence suggests the potential role of other circulating cells not clearly reported by the CellSearch® that could aid in the management of patients with BC.
Mesenchymal and epithelial-mesenchymal CTCs
EMT is an important phenomenon linked to increased invasiveness and resistance in tumor cells (70). Epithelial markers like EpCAM are lost during EMT, thus CTCs expressing only mesenchymal markers are missed using EpCAM-based enrichment methods. Studies using the CellSearch® system revealed the presence of cells expressing CK and lacking CD45 in the EpCAM-depleted fraction of roughly 70% blood samples and confirmed the malignant nature of these cells (71,72). Mesenchymal CTCs were reported also in samples with undetectable canonical CTCs, highlighting the potential additional information that the analysis of this subpopulation can provide. Additionally, CTCs can exhibit a mixed expression of epithelial and mesenchymal markers, indicating increased plasticity, a central feature in tumor progression and metastasis formation (73-76). A significant proportion (74–97%) of CK-positive CTCs in MBC patients also express mesenchymal markers, with lower prevalence in EBC, supporting the role of EMT in the metastatic potential of CTCs (77). Moreover, epithelial, mesenchymal and mixed CTCs may stem different biological processes. Yu et al. suggested an association of mesenchymal CTCs with disease progression (78). Bulfoni and colleagues observed that whereas epithelial and mixed epithelial-mesenchymal CTCs were associated with worse OS among patients with MBC, mesenchymal CTCs were associated with a better outcome (79). Other studies, supported the association of mixed CTCs with chemoresistance and poor outcomes in patients with MBC (80-82). In a study of MBC patients receiving eribulin-based treatment (N=22), total CTCs and mesenchymal CTCs were associated with PFS, while no difference in PFS was observed according to the epithelial CTC count (83). However, an updated analysis suggested that epithelial CTCs may be more important determinants of OS than EMT-CTCs (84). Whether the observed PFS difference is linked to eribulin’s effect on EMT suppression is yet unknown (85,86).
As for the early setting, Mego and colleagues found that EMT-CTCs were associated with poor prognosis in 427 patients with EBC, emphasizing their prognostic significance (87). A similar study based on gene expression analysis evaluated 83 EBC patients and showed that mesenchymal CTCs were characterized by the most aggressive phenotype, presence of lymph node metastases, larger tumor size, and seven-fold higher risk of death in the multivariable analysis (P=0.04) (88). In contrast, mixed epithelial-mesenchymal CTCs, believed to correspond to a highly plastic and aggressive state, did not show a significant impact on survival. A recent meta-analysis suggested that the prognostic value of EMT-CTCs might be more significant in patients with EBC compared to those with MBC (89).
Altogether these findings underscore the importance of evaluating mixed epithelial-mesenchymal and mesenchymal CTCs, as relying solely on epithelial markers might overlook a subset of patients at high risk for disease progression. Many CTC-detection approaches based on physical properties, including the FDA-approved Parsortix system (90), or on CD45-depletion have been developed to detect mesenchymal CTCs, providing additional tools for clinical assessment (91).
Cancer associated macrophage-like cells
Another population of circulating cells detected in BC patients is represented by cancer-associated macrophage-like cells (CAMLs) (92). CAMLs are defined as highly differentiated giant phagocytic cells (up to 300 µm) of myeloid lineage (CD14+/CD11c+) expressing EpCAM, CK but also CD45, characterized by large-atypical/multiple nuclei and by an oblong or amorphous shape. Using microfilters, CAMLs were isolated in 93% of BC patients, either non-metastatic or metastatic (average 19.4 cells per sample); of note, no CAMLs were detected in healthy subjects (93). Mu and colleagues evaluated the prognostic significance of CAMLs in MBC patients (N=127) using the CellSearch® System (94). At least one CAML was detected in 21 patients (16.5%) and associated with increased risk of disease progression (hazard ratio =1.75; 95% CI: 1.03–2.98; P=0.037) and death (hazard ratio =3.75; 95% CI: 1.52–9.26; P=0.004) in the multivariable analysis. Interestingly, when combining the presence of CAMLs and CTCs, patients with <5 CTCs and with CAMLs, with ≥5 CTCs but without CAMLs, or with ≥5 CTCs and with CAMLs, had an increasing trend of risk of disease progression and death as compared to patients with <5 CTCs and without CAMLs, (PFS: hazard ratio =0.84, 3.42, and 4.04 respectively, P<0.0001; OS: hazard ratio =2.66, 6.14, and 9.13, respectively, P<0.0001). Thus, the presence of CAMLs in peripheral blood might provide a potential biomarker with additional prognostic values over CTC enumeration alone in MBC patients.
Dual-positive cells (DPcells)
Another subpopulation of circulating cells worth further investigation is represented by DPcells which express both CD45 and epithelial markers (95). Studies in MBC patients have reported a wide range of DPcell detection (5–100%), mainly affected by the CTC-enrichment methods used (72,96,97). This subpopulation has been widely understudied so far since DPcells were detected also in healthy individuals, although at significantly lower levels compared to BC patients (96), and considered as false positive staining events (98). The origin and clinical meaning of these cells are still under investigation and in 2018, for the first time, a study on patients with pancreatic cancer suggested that DPcells are hybrid cells derived from the fusion of cancer cells with macrophages (99). Such fusion hybrids acquire macrophage-associated features endowing them with accelerated growth, increased motility, enhanced invasion activity, and thus a higher efficiency in metastasis formation. In the mentioned study, the presence of DPcells was also significantly associated with shorter OS, albeit the small cohort of patients (N=20) prevented definitive conclusions. By performing single-cell low-pass whole genome sequencing of DPcells collected from the blood of cancer patients (including BC) was demonstrated that a portion of DPcells have aberrant copy number aberration profiles (95), thus confirming their malignancy and reinforcing the heterotypic-cell fusion hypothesis. The fusion hypothesis is further supported by the observation that the majority of DPcells also express the macrophage marker CD68 (96). The association of circulating DPcells and OS was recently reported in a cohort of 341 BC patients supporting their tumor origin, with a prognostic impact observed primarily in patients with <5 CTCs and triple-negative BC (100). The fact that DPcells were present also in patients who do not present canonical CTCs (DPcells detected in 45% of samples of which 43% and 57% had ≥5 and <5 CTCs, respectively), raises new questions about their clinical relevance which should be further addressed, independently from their origin.
In summary, these studies highlight that beyond canonical CTCs other subpopulations of circulating rare cells, either tumor or stroma-derived cells, can be detected in the bloodstream and the notion of increased clinical utility through the utilization of pathological insights accompanying the identification of multiple rare circulating cell types simultaneously has been suggested (101). This multitype cell-based liquid biopsy approach would present a mosaic-like depiction of the underlying disease, thus offering a valuable tool for the management of patients with BC. Nevertheless, studies on these non-canonical cells are currently limited, and their clinical validity is not yet well-established. The primary limitation stems from the diverse technologies employed across studies, emphasizing the need for more standardized approaches to study this subpopulation and gain a clearer understanding of the mechanisms governing their presence and biological significance.
Circulating tumor RNAs (ctRNAs)
Recently, there has been increased research interest surrounding circulating noncoding RNAs (ncRNAs), which do not evolve into protein synthesis, as novel biomarkers in cancer (102). ncRNAs include a vast group of circulating oligonucleotides, such as long noncoding RNAs (lncRNAs), transfer RNAs (tRNAs), ribosomal RNAs (rRNAs), and small RNAs, such as microRNAs (miRNAs; short single-stranded noncoding RNAs of 20–25 nucleotides) and circular RNAs (circRNA), among many others. These molecules serve as regulators of gene transcription, exhibiting resistance to enzymatic cleavage in the blood (103).
Among various types of ncRNA, miRNAs have shown a major role in BC, acting as oncogenes (oncomiRNAs) or gene suppressors and playing an important role in cancer progression and oncogenic transformation (104,105). In the bloodstream, tumor-released miRNAs are protected from ubiquitous ribonuclease (RNase) enzyme-mediated degradation by embedding in exosomes or larger microvesicles shed from the plasma membrane. They also coexist as free-form ctRNA associated with a ribonucleoprotein complex or an argonaute-2 protein (106,107).
These non-exosomal free oligonucleotides have been shown to be involved in driving the progression of CTCs. From secondary analyses done on a cohort of 48 post-operative patients recruited in the frame of the SUCCESS A trial, miR-127 correlated with the presence of CTCs (108). Some miRNAs, particularly miR-199a-5p, have been shown to predict CTC clearance during treatment in patients with locally advanced BC exposed to neoadjuvant treatment, providing a molecular response assessment (109).
In a study conducted on an extended cohort of 269 individuals, a panel of circulating miRNAs (miR-141, miR-200a, miR-200b, miR-200c, miR-203, miR-210, miR-375, and miR-801) predicted the CTC status of patients with MBC and was able to differentiate cancer patients from healthy donors (110). In this research miR-200b was identified as the best marker to discriminate CTC-positive from negative patients.
Statistical evaluation of a model for OS and PFS prediction supported this finding showing that adding miR-200b to CTCs, compared with CTCs alone, significantly improved the model’s adherence to the data (likelihood-ratio test, P=0.002 for PFS and P<0.001 for OS) (111,112). In the study by Fischer et al., the circulating miR-200 family confirmed its role in BC and EMT, showing how increased levels of miR-200s and elevated CTC count correlated independently with poorer OS and PFS (112). However, combined data are not shown. The main limitation of this study is the small sample size and the use of the CellSearch® system, which is not sensitive in measuring CTCs that have undergone EMT.
Even though data regarding the prognostic role of miRNA are strong and rapidly expanding, data on the combined predictive and prognostic role of miRNA and CTCs are still limited and underpowered.
CTCs and circulating non-malignant cells
Considering the rising awareness about the key role of the tumor microenvironment and peripheral immunological processes, the role of blood analytes, though not directly derived from tumor cells, has gained importance. The various circulating blood cell ratios [neutrophil-to-lymphocyte ratio (NLR); monocyte-to-lymphocyte ratio (MLR); platelet-to-lymphocyte ratio (PLR)] could potentially serve as indicators to assess the immunological status, prognosis, and treatment outcomes (113,114). Monocytes and neutrophils, especially when considering the MLR and NLR index, have been associated with CTCs as prognostic indicators for OS in metastatic settings (115). In particular, MLR correlated with CTCs in triple-negative MBC, remaining significant also at the multivariable analysis.
Moreover, CTCs during the hematogenous dissemination can interact with different normal blood components such as platelets, neutrophils, monocytes, and endothelial cells to enhance their survival. Heterotypic clusters consist of not only CTCs but also immune cells, blood, and stromal cells associated with the tumor cells. Although these associated cells lack carcinogenic mutations, their phenotype undergoes changes that contribute to promoting tumor progression (116). It has been recently reported that red blood cells (RBCs) that accompany CTCs have prognostic value in MBC patients (117) and acquire modifications in their proteomic composition that were not observed among healthy donors (118). The lysosome-associated membrane glycoprotein 2 (LAMP2) was overexpressed in RBCs and high levels were associated with worse outcomes in MBC patients, suggesting that alterations in non-malignant components may provide additional information next to CTCs, though these are preliminary results and further studies are needed. The interaction between tumor cells and platelets has been demonstrated to modify the signaling pathways of platelets, referred to as tumor-educated platelets (TEP) (119). In research including various cancer types, TEP-derived RNA profiles, were used to successfully identify BC patients with an average accuracy of 62%, distinguishing also molecular subtypes (HER2+, PIK3CA, and triple-negative) (120). Data regarding the potential association between CTCs and TEP are lacking with wide room for further studies in the field.
Immunosuppression and smoldering-inflammation is a hallmark of BC with inflammatory circulating cells demonstrating to be involved in cancer progression and CTCs chaperoning (121). Macrophages and neutrophils can polarize into the pro-tumoral population.
In the work of the Aceto group, CTC-neutrophil clusters were associated with a more unfavorable prognosis in patients with MBC, as opposed to singular CTCs or CTC clusters (122). In the study, using single-cell RNA-sequencing (scRNA-seq) analysis, the researchers uncovered that CTC-myeloid clusters were predominantly composed of lymphocyte antigen 6 complex, locus G (LY6G) + neutrophils exhibiting a protumor ‘N2-like’ signature, indicative of a polarized state linked to immune suppression.
Findings concerning circulating cancer-associated cells exhibiting both epithelial and macrophage/myeloid characteristics provided backing for the hypothesis that fusion events between CTCs and pro-tumoral macrophages could play a crucial role in tumor progression. As previously said, CAMLs have been detected in the peripheral blood of patients with BC, showing to be able to provide prognostic information along with CTC detection (94). Circulating myeloid-derived suppressor cells (MDSCs) may be another heterogeneous immunosuppressive cell population correlating with CTCs and inducing worse survival outcomes in BC (123). High levels of monocytic-MDSCs tend to be related to a higher number of CTCs in patients with aggressive disease and de novo metastatic disease suggesting their role in the immunoregulatory switch responsible for cancer dissemination (124).
Few data regarding circulating endothelial cells (CECs) and CTCs suggested a potential correlation between them. Two studies, in the metastatic setting, showed CECs and CTCs to be independently correlated to a significantly worse outcome, however, the two counts did not result to be correlated with each other (125,126). A study conducted including EBC patients demonstrated a significantly poorer DFS in patients who experienced a rise in CEC/CTC counts ratio during adjuvant radiotherapy (P=0.004) (127).
Challenges of a multiparametric approach
All these data emphasize that the information depicted by a single blood analyte is limited and a multiparametric approach integrating information on different levels might be more informative and more comprehensively reflect the characteristics of the disease (Tables 1,2). However, along with opportunities, it is crucial to acknowledge and address the challenges that come with the implementation of such a holistic approach.
Table 1
Reference | Clinical purpose | Clinical setting | Analytes | Conclusions | |||
---|---|---|---|---|---|---|---|
CTCs | cfDNA/ctDNA | tdEVs | miRNA | ||||
Dawon et al. (15) | Monitoring | MBC | Count | Levels | Greater dynamic range and correlation with changes in tumor burden of ctDNA than CA 15-3 or CTC | ||
Prognosis | |||||||
Shaw et al. (24) | Prognosis | MBC | Count | Levels | High CTC count and total cfDNA level were significantly associated with poorer OS | ||
Ye et al. (25) | Prognosis | MBC | Count | Levels | CTC and total cfDNA levels were individually and jointly associated with PFS and OS | ||
Bortolini Silveira et al. (26) | Prognosis | HER2− MBC | Count | Level mutations | Both CTC and ctDNA levels were correlated with survival. KMT2C/MLL3 variants by ctDNA significantly associated with a lower CTC count; opposite trend was seen with GATA3 alterations | ||
Fernandez-Garcia et al. (20) | Prognosis | MBC | Count | Level | Both cfDNA and CTCs predictors of OS, only cfDNA predictor for PFS and disease response | ||
Therapy monitoring | |||||||
Gerratana et al. (27) | Longitudinal evolution | MBC | Count | Level (MAF) NOA | MAF trends reflected the treatment response. NOA steadily increased across time points. CTCs enumeration significantly increased only between first evaluation and progression | ||
Prognosis | |||||||
Radovich et al. (32) | Relapse prediction | TN EBC | Count | Detection | The positivity of both CTC and ctDNA had a negative impact on DDFS, DFS and OS | ||
Stergiopoulou et al. (33) | Relapse prediction | EBC | Count | Mutations | Positivity for at least one LB marker predictive of relapse. The molecular characteristics of CTCs were highly different at different time points, and always increased before the clinical relapse | ||
Phenotypic analysis | |||||||
Gene expression | |||||||
Methylation | |||||||
Davis et al. (38) | Analytic validity | MBC | Count | SNVs, CNVs, and gene fusions | CDKN2A correlated with lower number of CTC; while ESR1, GATA3, CDH1 and CCND1 correlated with higher number of CTC. CTC clusters were significantly associated with somatic genomic alterations in CDH1, CCND1, and BRCA1 | ||
Paolillo et al. (44) | Technical feasibility | HR+ MBC | ESR1 | ESR1 | Concordance of ESR1 mutations between ER+ CTC and cfDNA | ||
Beije et al. (45) | Therapy monitoring | HR+ MBC | ESR1 (hotspot mutations, splice variants) | ESR1 | The ESR1 mutations’ detection rate after ET therapy based on ctDNA and CTCs was 42% and 8%, respectively | ||
Tzanikou et al. (46) | Molecular characterization | EBC and MBC | PIK3CA (hotspot mutations) | PIK3CA | The detection and concordance of PIK3CA hotspot mutations between plasma-ctDNA and CTCs are higher in the metastatic setting | ||
Keup et al. (47) | Molecular characterization | HR+/HER2− MBC | Gene expression | Mutations | Most variants were unique in either ctDNA or CTCs; only 28% overlapped. PIK3CA and ESR1 variants were less common in CTC gDNA, while ERBB2 variants were only detected in CTC gDNA | ||
Chimonidou et al. (52) | Molecular characterization | EBC and MBC | Methylation | Methylation | High correlation in SOX17 promoter methylation in both early and MBC, with a negative prognostic impact on OS | ||
Prognosis | |||||||
Mastoraki et al. (54) | Therapy monitoring | HR+/HER2− MBC | Methylation | Methylation | Highly concordant ESR1 methylation in CTCs and corresponding ctDNA. ESR1 methylation in CTCs associated with a lack of response to everolimus-exemestane | ||
Nanou et al. (60) | Prognosis | MBC | Count | Count | Presence of unfavorable CTCs (cut-off >5) and tdEVs (cut-off ≥20) is predictive of OS. TdEVs can further stratify prognosis of patients with favorable CTC count | ||
Nanou et al. (61) | Prognosis | MBC | Count | Count | Elevated tdEV levels were independently associated with poorer OS. Complementary prognostic significance of tdEVs and CTCs | ||
Nanou et al. (62) | HER2 assessment | EBC and MBC | Count | Count | Inter- and intra-patient heterogeneity for the CTC/tdEV phenotypes associated with worse prognosis. The use of ≥7% HER2+CK+ tdEVs can predict HER2 expression of the tissue with 74% sensitivity and specificity using the HER2 amplification status of the primary tumor as a classification variable | ||
Prognosis | CK and HER2 phenotype | CK and HER2 phenotype | |||||
Keup et al. (68) | Therapy management | HR+/HER2− MBC | mRNA | mRNA | Concordance 5%. Divergent clinical outcomes were observed for mTOR transcript overexpression in CTCs versus EVs | ||
Keup et al. (131) | Longitudinal monitoring | HR+/HER2− MBC | mRNA | Mutations | mRNA | The presence of either ERBB3 signals in CTCs or EVs or cfDNA variants in ERBB3 showed a significant association with progressive MBC. The three analytes, each with their own unique features for disease monitoring, were shown to be complementary, underlining the usefulness of the longitudinal multi-parametric liquid biopsy approach | |
Keup et al. (130) | Clinical relevance | HR+/HER2− MBC | mRNA | Mutations | mRNA | A combination of two/three/four LBAs increased the prevalence of patients with actionable signals. Aggregating the results of hierarchical clustering of individual LBAs into the ELIMA score resulted in a highly significant correlation with OS | |
genomic DNA | |||||||
Alunni-Fabbroni et al. (108) | Associations of miRNA with CTC | EBC | Count | Concentration and characterization | Significant correlation between miR-200b and lymph node status and between miR-20a and tumor type. miR-127 correlated with the presence of CTCs. Borderline significant association between PFS and miR-19a levels | ||
Prognosis | |||||||
Akkiprik et al. (109) | Prognosis | EBC | Isolation and characterization for EMT, drug resistance and stemness markers | Isolation and characterization | miR-199a-5p predicts CTC clearance during treatment in patients with locally advanced BC exposed to neoadjuvant treatment, providing a molecular response assessment | ||
Madhavan et al. (110) | Prediction of the CTC status | MBC | Count | Profilation | A panel of circulating miRNAs (miR-141, miR-200a, miR-200b, miR-200c, miR-203, miR-210, miR-375, and miR-801) predicted the CTC status of patients with MBC and was able to differentiate cancer patients from healthy donors | ||
Fischer et al. (111) | Prognosis | MBC | Count | Expression level- miR-200 family | The levels of miR-200s were elevated in CTC-positive versus CTC-negative pts. Increased levels of miR-200s and elevated CTC count correlated independently with poorer OS and PFS |
Studies including circulating tumor cells and other non-cellular analytes (i.e., ctDNA, tdEVs, and miRNA) are described in the table, categorized for clinical purpose and setting. CTCs, circulating tumor cells; cfDNA, circulating free DNA; ctDNA, circulating tumor DNA; tdEVs, tumor-derived extracellular vesicles; miRNA, microRNAs; MBC, metastatic breast cancer; OS, overall survival; HER2, human epidermal growth factor receptor 2; MAF, mutant allele frequency; NOA, number of alterations; TN, triple-negative; EBC, early-stage breast cancer; DDFS, distant disease-free survival; DFS, disease-free survival; LB, liquid biopsy; SNVs, single-nucleotide variations; CNVs, copy number variations; HR+, hormone receptor positive; ER, estrogen receptor; mTOR, mammalian target of rapamycin; EVs, extracellular vesicles; LBAs, liquid biopsy analytes; PFS, progression-free survival; EMT, epithelial-to-mesenchymal transition.
Table 2
Reference | Clinical purpose | Clinical setting | Analytes | Analysis | Conclusions |
---|---|---|---|---|---|
Yu et al. (78) | Prognosis | MBC | Mesenchymal CTC | Count | Association of mesenchymal CTCs with disease progression |
Bulfoni et al. (79) | Clinicopathological features | MBC | E-CTC, EM-CTC, MES, NEG | Detection by DEPArray-based strategy | Significant association between specific CD45 neg subpopulations and tumor subtypes, proliferation, and sites of metastatic spread. EM-CTC was significantly associated with poorer PFS and OS |
Prognosis | |||||
Giordano et al. (80) | Characterization | HER2+ MBC | EM-CTC | Gene transcripts of EMT-TF and CSC features | HER2 + MBCs had EMT-CTCs. An enrichment of CSCs was found in CD326− and CD45− cells. EM-CTC related to lower PFS and OS |
Prognosis | |||||
Gradilone et al. (81) | Prognosis | MBC | Mesenchymal CTC | Cytokeratin and markers of epithelial mesenchymal transition expression | The presence of mesenchymal markers on CTC more accurately predicted worse prognosis than the expression of cytokeratin alone |
Papadaki et al. (82) | Prognosis | MBC | CSC+/partial-EMT+ CTCs | Cytokeratin expression | CSC+/partial-EMT+ CTCs correlated to lung metastases and decreased PFS. In HER2− patients related also to decreased OS and chemoresistance |
Horimoto et al. (83); Ito et al. (84) | Prognosis | MBC | EM-CTC | Count and characterization | Higher CTCs number and mesenchymal CTC associated with negative prognostic impact on eribulin’s PFS. Total CTCs number related to worse OS |
Mego et al. (87) | Prognosis | EBC | EM-CTC | Detection | EM-CTC have a negative prognostic value in primary BC |
Markiewicz et al. (88) | Clinical significance | EBC | CTCs with different EMT status | Isolation and molecular characterization | MES characterized by the most aggressive phenotype, lymph nodes metastases, larger tumor size and higher risk of death. EM-CTC no impact on survival |
Mu et al. (94) | Prognosis | MBC | CAMLs | Detection and count | CAMLs negative prognostic impact on PFS and OS. Combining with CTCs number, the presence of CAMLs with either <5 CTCs or >5 CTS had a negative prognostic impact on both PFS and OS, while only >5 CTCs and CAMLs—retained the statistical significance |
Reduzzi et al. (100) | Prognosis | EBC and MBC | DPcells | Detection and count | Prognostic impact observed primarily in patients with <5 CTCs and triple-negative BC |
De Giorgi et al. (115) | Correlation between CTC and inflammation based scores | MBC | Neutrophils, lymphocytes, platelets, monocytes | MLR, NLR, PLR, CTCs count | CTC and MLR are predictors of OS in MBC. CTC correlated with monocytes, in particular in triple-negative tumors |
Carmona-Ule et al. (117) | Prognosis | MBC | RBCs | CTC culture | The presence of RBCs in the culture was linked to a worse patient outcome |
Pereira-Veiga et al. (118) | Prognosis | EBC and MBC | RBCs | Proteomic composition | Different proteomic profile compared with healthy donors and between different tumor stages. Lysosome-associated membrane glycoprotein 2 emerge as a new RBCs marker with diagnostic and prognostic potential for MBC patients |
Best et al. (120) | Diagnostic potential | BC | TEPs | RNA-profile | TEP-derived RNA profiles, were used to successfully identify BC patients with an average accuracy of 62%, distinguishing also molecular subtypes (HER2+, PIK3CA, and triple-negative) |
Mego et al. (121) | Correlation between CTC and PB-T cell | IBC | PB T-cell | CTC enumeration, flow cytometry for T cell phenotype and function | IBC patients with CTCs in PB had abnormalities in adaptive immunity that could potentially impact tumor cell dissemination and initiation of the metastatic cascade |
Szczerba et al. (122) | Identification and function | BC | CTC-associated WBCs | Isolation and characterization | CTC-neutrophil clusters were associated with a more unfavorable prognosis in patients with MBC, as opposed to singular CTCs or CTC clusters |
Cole et al. (123) | Correlation with CTCs and prognosis | MBC | MDSCs | Enumeration | MDSCs correlated with CTCs and were associated with overall survival |
Bergenfelz et al. (124) | Clinical significance | MBC | Monocytic-MDSCs | Flow cytometric analysis | High levels of monocytic-MDSCs tend to be related to a higher number of CTCs in patients with aggressive disease and de novo metastatic disease suggesting their role in the immunoregulatory switch responsible for cancer dissemination |
Bidard et al. (125) | Prognosis | MBC | CECs | Count | CTC and CECs independent prognostic factor. Increase in CEC count was associated with improved time to progression, at the threshold of 20 CECs/4 mL |
Codes et al. (126) | Therapy monitoring | HER2− MBC | CECs | Count | CTC and CECs independent prognostic factor. Baseline CECs >200 was associated with lower PFS |
Mäurer et al. (127) | Disease relapse | EBC | CECs/CTC | Count | Patients with an increase in CETC/CTC numbers over the course of adjuvant RT had a significantly worse disease-free survival than patients with stable or decreasing CETC/CTC numbers |
Studies including circulating tumor cells and other cellular analytes are described in the table, categorized for clinical purpose and setting. CTCs, circulating tumor cells; MBC, metastatic breast cancer; E-CTC, cells expressing only epithelial markers; EM-CTC, epithelial-to-mesenchymal transition circulating tumor cell; MES, cells expressing only mesenchymal markers; NEG, cells negative for every tested marker; PFS, progression-free survival; OS, overall survival; HER2, human epidermal growth factor receptor 2; EMT-TF, epithelial-to-mesenchymal transition-inducing transcription factors; CSC, cancer steam cell; EBC, early-stage breast cancer; BC, breast cancer; EMT, epithelial-to-mesenchymal transition; CAMLs, cancer-associated macrophage-like cells; DPcells, dual-positive cells; MLR, monocyte-to-lymphocyte ratio; NLR, neutrophil-to-lymphocyte ratio; PLR, platelet-to-lymphocyte ratio; RBCs, red blood cells; TEPs, tumor-educated platelets; IBC, inflammatory breast cancer; PB, peripheral blood; WBCs, white blood cells; MDSCs, myeloid-derived suppressor cells; CECs, circulating endothelial cells; CETC, circulating epithelial tumor cell.
First, pre-analytical issues related to the collection, storage, and preparation of blood samples require shared validated protocols (128). Indeed analysis of different circulating biomarkers is based on different isolation protocols and can require different preservative blood collection tubes for a reliable analysis (129). Thus, blood volume needed for multiple analyses is additive and can hamper patients’ compliance. Workflows have been implemented to enable the parallel isolation and analysis of multiple biomarkers minimizing the blood volume. The ELIMA (Evaluation of multiple Liquid biopsy analytes In Metastatic breast cancer patients All from one blood sample) multiparametric approach included the analysis of EV mRNA, CTC mRNA, CTC genomic DNA, and cfDNA from CTC-depleted blood from 18 mL of EDTA blood (130). The integration of these four analytes enabled to simultaneously analyze the transcriptional and genomic complexity of BC and revealed improved sensitivity for actionable markers and prognostication. Another work from the same group showed the usefulness of a longitudinal multiparametric approach, combining CTC mRNA, EV mRNA and ctDNA, for disease monitoring in patients with HR-positive/HER2-negative MBC (131). A study in prostate cancer patients showed the feasibility of a combinational analysis of transcripts in CTCs, the same transcripts in whole blood, and focal amplifications in cfDNA from 12.5 mL of blood (132). This approach led to an increase in the probability of identifying resistance mechanisms as compared to the use of a single analyte (89% vs. 50%).
Furthermore, the standardization of liquid biopsy methodologies and the establishment of universally accepted protocols pose significant challenges. The variability in techniques and platforms across different studies hinders the seamless comparison of results and the translation of findings into clinical practice. Achieving a consensus on the standardization of liquid biopsy procedures is imperative to ensure the reproducibility and reliability of results across diverse clinical settings. The necessity of detecting a broader range of circulating tumor-derived material has led to the development of next generation systems. Among these, the third-generation high-definition single-cell assay (HDSCA3.0) workflow provides the ability to identify and characterize epithelial, mesenchymal, endothelial, and hematopoietic cells, as well as large EVs. A study demonstrated that, beyond CTCs, assessing a wider range of circulating events enhanced the ability to categorize normal donors, EBC, and MBC patients into distinct groups (133).
Another significant challenge pertains to the complexity of interpreting and integrating the vast array of information gathered from different circulating biomarkers. Each biomarker contributes unique insights into tumor biology, and navigating the interplay among these various components demands sophisticated analytical approaches. The challenge lies in harmonizing these diverse data streams to derive meaningful and actionable conclusions that can guide clinical decision-making. In this context, there is increasing interest in using combinatorial approaches driven by machine learning to increase sensitivity and accuracy. A multianalyte panel consisting of tumor-associated EV miRNAs and mRNAs, cfDNA, and CA19-9, demonstrated utility for early diagnosis and staging of pancreatic cancer in 204 subjects (134). Interestingly, in this study the investigators developed an initial classification model using 14 biomarker candidates and then trained a machine-learning model achieving a sensitivity of 88% and a specificity of 95%.
Finally, prospective, multicenter clinical trials including large cohorts of patients are needed to provide strong evidence of the clinical utility of multiparametric testing.
Conclusions
In conclusion, it appears evident that the diversity of analytes that can be obtained from the blood of patients with BC is remarkable, each presenting limitations and advantages. So far, CTC and ctDNA have been the most commonly utilized liquid biopsy biomarkers. A combined approach leveraging the strength of each, such as the heightened sensitivity of ctDNA and the ability of CTCs to offer insight into tumor biology, hold promise for improving early detection, prognostication, and treatment. Furthermore, integrating information from various circulating component, beyond CTCs and ctDNA, could yield an even more comprehensive disease profile. While these diverse analytes may be partially linked to CTCs, they mostly do not overlap, representing distinct biological processes, such as intercellular communication for EVs, or interaction with immune cells for CAMLs and DPcells. The fact that different circulating biomarker should be viewed as complementary/synergistic rather than competitive is becoming increasingly evident, not only in BC but also in other cancer types (135). While the comprehensive liquid biopsy approach opens up new opportunities in BC research and management, it is essential to address the associated challenges. Overcoming these hurdles will necessitate concerted efforts in refining detection methods, establishing standardized protocols, and developing analytical frameworks for data integration. As the field continues to evolve, these endeavors will be instrumental for unlocking the full potential of liquid biopsy in revolutionizing our understanding and approach to BC.
Acknowledgments
Funding: This work was supported by
Footnote
Peer Review File: Available at https://tbcr.amegroups.org/article/view/10.21037/tbcr-23-55/prf
Conflicts of Interest: All authors have completed the ICMJE uniform disclosure form (available at https://tbcr.amegroups.org/article/view/10.21037/tbcr-23-55/coif). E.N. is supported by an American-Italian Cancer Foundation Post-Doctoral Research Fellowship. G.C. reports payment or honoraria for lectures, presentations, speaker bureaus, manuscript writing or educational events: Lilly, Pfizer, Relay, Gilead, Novartis; Consulting fees: BMS, Roche, Pfizer, Novartis, Lilly, Astra Zeneca, Daichii Sankyo, Merck, Seagen, Ellipsis, Gilead, Menarini; Grants or contracts: Merck; Support for attending meetings and/or travel: Daichii Sankyo. Institutional research funding for conducting Phase 1 and 2 clinical trials: Pfizer, Roche, Novartis, Sanofi, Celgene, Servier, Orion, AstraZeneca, Seattle Genetics, AbbVie, Tesaro, BMS, Merck Serono, Merck Sharp Dohme, Janssen-Cilag, Philogen, Bayer, Medivation, Medimmune. All outside the present work. M.C. reports personal fees from Lilly, Sermonix, Data Genomics, Foundation Medicine, Guardant Health, Celcuity, Iylon, and Ellipses and grants and personal fees from Pfizer, AZ and Menarini, all outside the submitted work. G.C. serves as an unpaid editorial board member of Translational Breast Cancer Research from March 2023 to February 2025. M.C. serves as an unpaid editorial board member of Translational Breast Cancer Research from April 2023 to March 2025. The other authors have no conflicts of interest to declare..
Ethical Statement: The authors are accountable for all aspects of the work in ensuring that questions related to the accuracy or integrity of any part of the work are appropriately investigated and resolved.
Open Access Statement: This is an Open Access article distributed in accordance with the Creative Commons Attribution-NonCommercial-NoDerivs 4.0 International License (CC BY-NC-ND 4.0), which permits the non-commercial replication and distribution of the article with the strict proviso that no changes or edits are made and the original work is properly cited (including links to both the formal publication through the relevant DOI and the license). See: https://creativecommons.org/licenses/by-nc-nd/4.0/.
References
- Freitas AJA, Causin RL, Varuzza MB, et al. Liquid Biopsy as a Tool for the Diagnosis, Treatment, and Monitoring of Breast Cancer. Int J Mol Sci 2022;23:9952. [Crossref] [PubMed]
- Siravegna G, Marsoni S, Siena S, et al. Integrating liquid biopsies into the management of cancer. Nat Rev Clin Oncol 2017;14:531-48. [Crossref] [PubMed]
- Pantel K, Alix-Panabières C. Circulating tumour cells in cancer patients: challenges and perspectives. Trends Mol Med 2010;16:398-406. [Crossref] [PubMed]
- Allard WJ, Matera J, Miller MC, et al. Tumor cells circulate in the peripheral blood of all major carcinomas but not in healthy subjects or patients with nonmalignant diseases. Clin Cancer Res 2004;10:6897-904. [Crossref] [PubMed]
- Cani AK, Hayes DF. Breast Cancer Circulating Tumor Cells: Current Clinical Applications and Future Prospects. Clin Chem 2024;70:68-80. [Crossref] [PubMed]
- Bidard FC, Kiavue N, Jacot W, et al. Overall Survival With Circulating Tumor Cell Count-Driven Choice of Therapy in Advanced Breast Cancer: A Randomized Trial. J Clin Oncol 2024;42:383-9. [Crossref] [PubMed]
- Nicolò E, Serafini MS, Munoz-Arcos L, et al. Real-time assessment of HER2 status in circulating tumor cells of breast cancer patients: Methods of detection and clinical implications. J Liq Biopsy 2023;2:100117. [Crossref]
- Hayes DF, Cristofanilli M, Budd GT, et al. Circulating tumor cells at each follow-up time point during therapy of metastatic breast cancer patients predict progression-free and overall survival. Clin Cancer Res 2006;12:4218-24. [Crossref] [PubMed]
- Cristofanilli M, Budd GT, Ellis MJ, et al. Circulating tumor cells, disease progression, and survival in metastatic breast cancer. N Engl J Med 2004;351:781-91. [Crossref] [PubMed]
- Lucci A, Hall CS, Lodhi AK, et al. Circulating tumour cells in non-metastatic breast cancer: a prospective study. Lancet Oncol 2012;13:688-95. [Crossref] [PubMed]
- Bidard FC, Michiels S, Riethdorf S, et al. Circulating Tumor Cells in Breast Cancer Patients Treated by Neoadjuvant Chemotherapy: A Meta-analysis. J Natl Cancer Inst 2018;110:560-7. [Crossref] [PubMed]
- Keup C, Kimmig R, Kasimir-Bauer S. The Diversity of Liquid Biopsies and Their Potential in Breast Cancer Management. Cancers (Basel) 2023;15:5463. [Crossref] [PubMed]
- Schwarzenbach H, Hoon DS, Pantel K. Cell-free nucleic acids as biomarkers in cancer patients. Nat Rev Cancer 2011;11:426-37. [Crossref] [PubMed]
- Agostinetto E, Nader-Marta G, Ignatiadis M. Circulating tumor DNA in breast cancer: a biomarker for patient selection. Curr Opin Oncol 2023;35:426-35. [Crossref] [PubMed]
- Dawson SJ, Tsui DW, Murtaza M, et al. Analysis of circulating tumor DNA to monitor metastatic breast cancer. N Engl J Med 2013;368:1199-209. [Crossref] [PubMed]
- Yang J, Cheng L, Zhang J, et al. Predictive value of circulating cell-free DNA in the survival of breast cancer patients: A systemic review and meta-analysis. Medicine (Baltimore) 2018;97:e11417. [Crossref] [PubMed]
- Lee JH, Jeong H, Choi JW, et al. Liquid biopsy prediction of axillary lymph node metastasis, cancer recurrence, and patient survival in breast cancer: A meta-analysis. Medicine (Baltimore) 2018;97:e12862. [Crossref] [PubMed]
- Kong SL, Liu X, Tan SJ, et al. Complementary Sequential Circulating Tumor Cell (CTC) and Cell-Free Tumor DNA (ctDNA) Profiling Reveals Metastatic Heterogeneity and Genomic Changes in Lung Cancer and Breast Cancer. Front Oncol 2021;11:698551. [Crossref] [PubMed]
- Wang W, Liang M, Ma G, et al. Plasma cell-free DNA integrity plus circulating tumor cells: a potential biomarker of no distant metastasis breast cancer. Neoplasma 2017;64:611-8. [Crossref] [PubMed]
- Fernandez-Garcia D, Hills A, Page K, et al. Plasma cell-free DNA (cfDNA) as a predictive and prognostic marker in patients with metastatic breast cancer. Breast Cancer Res 2019;21:149. [Crossref] [PubMed]
- Rossi G, Mu Z, Rademaker AW, et al. Cell-Free DNA and Circulating Tumor Cells: Comprehensive Liquid Biopsy Analysis in Advanced Breast Cancer. Clin Cancer Res 2018;24:560-8. [Crossref] [PubMed]
- Suppan C, Brcic I, Tiran V, et al. Untargeted Assessment of Tumor Fractions in Plasma for Monitoring and Prognostication from Metastatic Breast Cancer Patients Undergoing Systemic Treatment. Cancers (Basel) 2019;11:1171. [Crossref] [PubMed]
- Madic J, Kiialainen A, Bidard F, et al. Circulating tumor DNA and circulating tumor cells in metastatic triple negative breast cancer patients. Int J Cancer 2015;136:2158-65. [Crossref] [PubMed]
- Shaw JA, Guttery DS, Hills A, et al. Mutation Analysis of Cell-Free DNA and Single Circulating Tumor Cells in Metastatic Breast Cancer Patients with High Circulating Tumor Cell Counts. Clin Cancer Res 2017;23:88-96. [Crossref] [PubMed]
- Ye Z, Wang C, Wan S, et al. Association of clinical outcomes in metastatic breast cancer patients with circulating tumour cell and circulating cell-free DNA. Eur J Cancer 2019;106:133-43. [Crossref] [PubMed]
- Bortolini Silveira A, Bidard FC, Tanguy ML, et al. Multimodal liquid biopsy for early monitoring and outcome prediction of chemotherapy in metastatic breast cancer. NPJ Breast Cancer 2021;7:115. [Crossref] [PubMed]
- Gerratana L, Davis AA, Zhang Q, et al. Longitudinal Dynamics of Circulating Tumor Cells and Circulating Tumor DNA for Treatment Monitoring in Metastatic Breast Cancer. JCO Precis Oncol 2021;5:943-52. [Crossref] [PubMed]
- Garcia-Murillas I, Schiavon G, Weigelt B, et al. Mutation tracking in circulating tumor DNA predicts relapse in early breast cancer. Sci Transl Med 2015;7:302ra133. [Crossref] [PubMed]
- Riva F, Bidard FC, Houy A, et al. Patient-Specific Circulating Tumor DNA Detection during Neoadjuvant Chemotherapy in Triple-Negative Breast Cancer. Clin Chem 2017;63:691-9. [Crossref] [PubMed]
- McDonald BR, Contente-Cuomo T, Sammut SJ, et al. Personalized circulating tumor DNA analysis to detect residual disease after neoadjuvant therapy in breast cancer. Sci Transl Med 2019;11:eaax7392. [Crossref] [PubMed]
- Li S, Lai H, Liu J, et al. Circulating Tumor DNA Predicts the Response and Prognosis in Patients With Early Breast Cancer Receiving Neoadjuvant Chemotherapy. JCO Precis Oncol 2020;4:PO.19.00292.
- Radovich M, Jiang G, Hancock BA, et al. Association of Circulating Tumor DNA and Circulating Tumor Cells After Neoadjuvant Chemotherapy With Disease Recurrence in Patients With Triple-Negative Breast Cancer: Preplanned Secondary Analysis of the BRE12-158 Randomized Clinical Trial. JAMA Oncol 2020;6:1410-5. [Crossref] [PubMed]
- Stergiopoulou D, Markou A, Strati A, et al. Comprehensive liquid biopsy analysis as a tool for the early detection of minimal residual disease in breast cancer. Sci Rep 2023;13:1258. [Crossref] [PubMed]
- Garcia-Murillas I, Chopra N, Comino-Méndez I, et al. Assessment of Molecular Relapse Detection in Early-Stage Breast Cancer. JAMA Oncol 2019;5:1473-8. [Crossref] [PubMed]
- Racila E, Euhus D, Weiss AJ, et al. Detection and characterization of carcinoma cells in the blood. Proc Natl Acad Sci U S A 1998;95:4589-94. [Crossref] [PubMed]
- Stecklein SR, Kimler BF, Yoder R, et al. ctDNA and residual cancer burden are prognostic in triple-negative breast cancer patients with residual disease. NPJ Breast Cancer 2023;9:10. [Crossref] [PubMed]
- Serafini MS, Molteni E, Nicolò E, et al. Cellular residual disease (CRD) in early breast cancer –Expanding the concept of minimal residual disease monitoring? J Liq Biopsy 2023;3:100132. [Crossref]
- Davis AA, Zhang Q, Gerratana L, et al. Association of a novel circulating tumor DNA next-generating sequencing platform with circulating tumor cells (CTCs) and CTC clusters in metastatic breast cancer. Breast Cancer Res 2019;21:137. [Crossref] [PubMed]
- Kouros-Mehr H, Bechis SK, Slorach EM, et al. GATA-3 links tumor differentiation and dissemination in a luminal breast cancer model. Cancer Cell 2008;13:141-52. [Crossref] [PubMed]
- Cho SJ, Yoon C, Lee JH, et al. KMT2C Mutations in Diffuse-Type Gastric Adenocarcinoma Promote Epithelial-to-Mesenchymal Transition. Clin Cancer Res 2018;24:6556-69. [Crossref] [PubMed]
- Gala K, Li Q, Sinha A, et al. KMT2C mediates the estrogen dependence of breast cancer through regulation of ERα enhancer function. Oncogene 2018;37:4692-710. [Crossref] [PubMed]
- Shishido SN, Masson R, Xu L, et al. Disease characterization in liquid biopsy from HER2-mutated, non-amplified metastatic breast cancer patients treated with neratinib. NPJ Breast Cancer 2022;8:22. [Crossref] [PubMed]
- Welter L, Xu L, McKinley D, et al. Treatment response and tumor evolution: lessons from an extended series of multianalyte liquid biopsies in a metastatic breast cancer patient. Cold Spring Harb Mol Case Stud 2020;6:a005819. [Crossref] [PubMed]
- Paolillo C, Mu Z, Rossi G, et al. Detection of Activating Estrogen Receptor Gene (ESR1) Mutations in Single Circulating Tumor Cells. Clin Cancer Res 2017;23:6086-93. [Crossref] [PubMed]
- Beije N, Sieuwerts AM, Kraan J, et al. Estrogen receptor mutations and splice variants determined in liquid biopsies from metastatic breast cancer patients. Mol Oncol 2018;12:48-57. [Crossref] [PubMed]
- Tzanikou E, Markou A, Politaki E, et al. PIK3CA hotspot mutations in circulating tumor cells and paired circulating tumor DNA in breast cancer: a direct comparison study. Mol Oncol 2019;13:2515-30. [Crossref] [PubMed]
- Keup C, Storbeck M, Hauch S, et al. Multimodal Targeted Deep Sequencing of Circulating Tumor Cells and Matched Cell-Free DNA Provides a More Comprehensive Tool to Identify Therapeutic Targets in Metastatic Breast Cancer Patients. Cancers (Basel) 2020;12:1084. [Crossref] [PubMed]
- Song CX, Yin S, Ma L, et al. 5-Hydroxymethylcytosine signatures in cell-free DNA provide information about tumor types and stages. Cell Res 2017;27:1231-42. [Crossref] [PubMed]
- Gianni C, Palleschi M, Merloni F, et al. Cell-Free DNA Fragmentomics: A Promising Biomarker for Diagnosis, Prognosis and Prediction of Response in Breast Cancer. Int J Mol Sci 2022;23:14197. [Crossref] [PubMed]
- Jiang P, Sun K, Peng W, et al. Plasma DNA End-Motif Profiling as a Fragmentomic Marker in Cancer, Pregnancy, and Transplantation. Cancer Discov 2020;10:664-73. [Crossref] [PubMed]
- Chandrananda D, Thorne NP, Bahlo M. High-resolution characterization of sequence signatures due to non-random cleavage of cell-free DNA. BMC Med Genomics 2015;8:29. [Crossref] [PubMed]
- Chimonidou M, Strati A, Malamos N, et al. Direct comparison study of DNA methylation markers in EpCAM-positive circulating tumour cells, corresponding circulating tumour DNA, and paired primary tumours in breast cancer. Oncotarget 2017;8:72054-68. [Crossref] [PubMed]
- Chimonidou M, Strati A, Malamos N, et al. SOX17 promoter methylation in circulating tumor cells and matched cell-free DNA isolated from plasma of patients with breast cancer. Clin Chem 2013;59:270-9. [Crossref] [PubMed]
- Mastoraki S, Strati A, Tzanikou E, et al. ESR1 Methylation: A Liquid Biopsy-Based Epigenetic Assay for the Follow-up of Patients with Metastatic Breast Cancer Receiving Endocrine Treatment. Clin Cancer Res 2018;24:1500-10. [Crossref] [PubMed]
- Goberdhan DCI. Large tumour-derived extracellular vesicles as prognostic indicators of metastatic cancer patient survival. Br J Cancer 2023;128:471-3. [Crossref] [PubMed]
- Hilton SH, White IM. Advances in the analysis of single extracellular vesicles: A critical review. Sens Actuators Rep 2021;3:100052. [Crossref] [PubMed]
- Crescitelli R, Lässer C, Lötvall J. Isolation and characterization of extracellular vesicle subpopulations from tissues. Nat Protoc 2021;16:1548-80. [Crossref] [PubMed]
- Tian Y, Gong M, Hu Y, et al. Quality and efficiency assessment of six extracellular vesicle isolation methods by nano-flow cytometry. J Extracell Vesicles 2019;9:1697028. [Crossref] [PubMed]
- Nanou A, Coumans FAW, van Dalum G, et al. Circulating tumor cells, tumor-derived extracellular vesicles and plasma cytokeratins in castration-resistant prostate cancer patients. Oncotarget 2018;9:19283-93. [Crossref] [PubMed]
- Nanou A, Miller MC, Zeune LL, et al. Tumour-derived extracellular vesicles in blood of metastatic cancer patients associate with overall survival. Br J Cancer 2020;122:801-11. [Crossref] [PubMed]
- Nanou A, Miao J, Coumans FAW, et al. Tumor-Derived Extracellular Vesicles as Complementary Prognostic Factors to Circulating Tumor Cells in Metastatic Breast Cancer. JCO Precis Oncol 2023;7:e2200372. [Crossref] [PubMed]
- Nanou A, Zeune LL, Bidard FC, et al. HER2 expression on tumor-derived extracellular vesicles and circulating tumor cells in metastatic breast cancer. Breast Cancer Res 2020;22:86. [Crossref] [PubMed]
- Isebia KT, Dathathri E, Verschoor N, et al. Characterizing Circulating Tumor Cells and Tumor-Derived Extracellular Vesicles in Metastatic Castration-Naive and Castration-Resistant Prostate Cancer Patients. Cancers (Basel) 2022;14:4404. [Crossref] [PubMed]
- Jiang Y, Palma JF, Agus DB, et al. Detection of androgen receptor mutations in circulating tumor cells in castration-resistant prostate cancer. Clin Chem 2010;56:1492-5. [Crossref] [PubMed]
- Marchetti A, Del Grammastro M, Felicioni L, et al. Assessment of EGFR mutations in circulating tumor cell preparations from NSCLC patients by next generation sequencing: toward a real-time liquid biopsy for treatment. PLoS One 2014;9:e103883. [Crossref] [PubMed]
- Crespo M, van Dalum G, Ferraldeschi R, et al. Androgen receptor expression in circulating tumour cells from castration-resistant prostate cancer patients treated with novel endocrine agents. Br J Cancer 2015;112:1166-74. [Crossref] [PubMed]
- Vagner T, Spinelli C, Minciacchi VR, et al. Large extracellular vesicles carry most of the tumour DNA circulating in prostate cancer patient plasma. J Extracell Vesicles 2018;7:1505403. [Crossref] [PubMed]
- Keup C, Mach P, Aktas B, et al. RNA Profiles of Circulating Tumor Cells and Extracellular Vesicles for Therapy Stratification of Metastatic Breast Cancer Patients. Clin Chem 2018;64:1054-62. [Crossref] [PubMed]
- König L, Kasimir-Bauer S, Bittner AK, et al. Elevated levels of extracellular vesicles are associated with therapy failure and disease progression in breast cancer patients undergoing neoadjuvant chemotherapy. Oncoimmunology 2017;7:e1376153. [Crossref] [PubMed]
- Dongre A, Weinberg RA. New insights into the mechanisms of epithelial-mesenchymal transition and implications for cancer. Nat Rev Mol Cell Biol 2019;20:69-84. [Crossref] [PubMed]
- de Wit S, Manicone M, Rossi E, et al. EpCAM(high) and EpCAM(low) circulating tumor cells in metastatic prostate and breast cancer patients. Oncotarget 2018;9:35705-16. [Crossref] [PubMed]
- Schneck H, Gierke B, Uppenkamp F, et al. EpCAM-Independent Enrichment of Circulating Tumor Cells in Metastatic Breast Cancer. PLoS One 2015;10:e0144535. [Crossref] [PubMed]
- Tsai JH, Donaher JL, Murphy DA, et al. Spatiotemporal regulation of epithelial-mesenchymal transition is essential for squamous cell carcinoma metastasis. Cancer Cell 2012;22:725-36. [Crossref] [PubMed]
- Tsuji T, Ibaragi S, Shima K, et al. Epithelial-mesenchymal transition induced by growth suppressor p12CDK2-AP1 promotes tumor cell local invasion but suppresses distant colony growth. Cancer Res 2008;68:10377-86. [Crossref] [PubMed]
- Ocaña OH, Córcoles R, Fabra A, et al. Metastatic colonization requires the repression of the epithelial-mesenchymal transition inducer Prrx1. Cancer Cell 2012;22:709-24. [Crossref] [PubMed]
- Brabletz T. EMT and MET in metastasis: where are the cancer stem cells? Cancer Cell 2012;22:699-701. [Crossref] [PubMed]
- Kallergi G, Papadaki MA, Politaki E, et al. Epithelial to mesenchymal transition markers expressed in circulating tumour cells of early and metastatic breast cancer patients. Breast Cancer Res 2011;13:R59. [Crossref] [PubMed]
- Yu M, Bardia A, Wittner BS, et al. Circulating breast tumor cells exhibit dynamic changes in epithelial and mesenchymal composition. Science 2013;339:580-4. [Crossref] [PubMed]
- Bulfoni M, Gerratana L, Del Ben F, et al. In patients with metastatic breast cancer the identification of circulating tumor cells in epithelial-to-mesenchymal transition is associated with a poor prognosis. Breast Cancer Res 2016;18:30. [Crossref] [PubMed]
- Giordano A, Gao H, Anfossi S, et al. Epithelial-mesenchymal transition and stem cell markers in patients with HER2-positive metastatic breast cancer. Mol Cancer Ther 2012;11:2526-34. [Crossref] [PubMed]
- Gradilone A, Raimondi C, Nicolazzo C, et al. Circulating tumour cells lacking cytokeratin in breast cancer: the importance of being mesenchymal. J Cell Mol Med 2011;15:1066-70. [Crossref] [PubMed]
- Papadaki MA, Stoupis G, Theodoropoulos PA, et al. Circulating Tumor Cells with Stemness and Epithelial-to-Mesenchymal Transition Features Are Chemoresistant and Predictive of Poor Outcome in Metastatic Breast Cancer. Mol Cancer Ther 2019;18:437-47. [Crossref] [PubMed]
- Horimoto Y, Tokuda E, Murakami F, et al. Analysis of circulating tumour cell and the epithelial mesenchymal transition (EMT) status during eribulin-based treatment in 22 patients with metastatic breast cancer: a pilot study. J Transl Med 2018;16:287. [Crossref] [PubMed]
- Ito M, Horimoto Y, Tokuda E, et al. Impact of circulating tumour cells on survival of eribulin-treated patients with metastatic breast cancer. Med Oncol 2019;36:89. [Crossref] [PubMed]
- Dybdal-Hargreaves NF, Risinger AL, Mooberry SL. Regulation of E-cadherin localization by microtubule targeting agents: rapid promotion of cortical E-cadherin through p130Cas/Src inhibition by eribulin. Oncotarget 2018;9:5545-61. [Crossref] [PubMed]
- Yoshida T, Ozawa Y, Kimura T, et al. Eribulin mesilate suppresses experimental metastasis of breast cancer cells by reversing phenotype from epithelial-mesenchymal transition (EMT) to mesenchymal-epithelial transition (MET) states. Br J Cancer 2014;110:1497-505. [Crossref] [PubMed]
- Mego M, Karaba M, Minarik G, et al. Circulating Tumor Cells With Epithelial-to-mesenchymal Transition Phenotypes Associated With Inferior Outcomes in Primary Breast Cancer. Anticancer Res 2019;39:1829-37. [Crossref] [PubMed]
- Markiewicz A, Topa J, Nagel A, et al. Spectrum of Epithelial-Mesenchymal Transition Phenotypes in Circulating Tumour Cells from Early Breast Cancer Patients. Cancers (Basel) 2019;11:59. [Crossref] [PubMed]
- Zhao Q, Li B, Gao Q, et al. Prognostic value of epithelial-mesenchymal transition circulating tumor cells in female breast cancer: A meta-analysis. Front Oncol 2022;12:1024783. [Crossref] [PubMed]
- Cohen EN, Jayachandran G, Moore RG, et al. A Multi-Center Clinical Study to Harvest and Characterize Circulating Tumor Cells from Patients with Metastatic Breast Cancer Using the Parsortix(®) PC1 System. Cancers (Basel) 2022;14:5238. [Crossref] [PubMed]
- Batth IS, Mitra A, Rood S, et al. CTC analysis: an update on technological progress. Transl Res 2019;212:14-25. [Crossref] [PubMed]
- Adams DL, Martin SS, Alpaugh RK, et al. Circulating giant macrophages as a potential biomarker of solid tumors. Proc Natl Acad Sci U S A 2014;111:3514-9. [Crossref] [PubMed]
- Adams DL, Adams DK, Alpaugh RK, et al. Circulating Cancer-Associated Macrophage-Like Cells Differentiate Malignant Breast Cancer and Benign Breast Conditions. Cancer Epidemiol Biomarkers Prev 2016;25:1037-42. [Crossref] [PubMed]
- Mu Z, Wang C, Ye Z, et al. Prognostic values of cancer associated macrophage-like cells (CAML) enumeration in metastatic breast cancer. Breast Cancer Res Treat 2017;165:733-41. [Crossref] [PubMed]
- Reduzzi C, Vismara M, Gerratana L, et al. The curious phenomenon of dual-positive circulating cells: Longtime overlooked tumor cells. Semin Cancer Biol 2020;60:344-50. [Crossref] [PubMed]
- Lustberg MB, Balasubramanian P, Miller B, et al. Heterogeneous atypical cell populations are present in blood of metastatic breast cancer patients. Breast Cancer Res 2014;16:R23. [Crossref] [PubMed]
- Khoo BL, Warkiani ME, Tan DS, et al. Clinical validation of an ultra high-throughput spiral microfluidics for the detection and enrichment of viable circulating tumor cells. PLoS One 2014;9:e99409. [Crossref] [PubMed]
- Fischer JC, Niederacher D, Topp SA, et al. Diagnostic leukapheresis enables reliable detection of circulating tumor cells of nonmetastatic cancer patients. Proc Natl Acad Sci U S A 2013;110:16580-5. [Crossref] [PubMed]
- Gast CE, Silk AD, Zarour L, et al. Cell fusion potentiates tumor heterogeneity and reveals circulating hybrid cells that correlate with stage and survival. Sci Adv 2018;4:eaat7828. [Crossref] [PubMed]
- Reduzzi C, Gerratana L, Zhang Y, et al. CK+/CD45+ (dual-positive) circulating cells are associated with prognosis in patients with advanced breast cancer. J Clin Oncol 2022;40:1093. [Crossref]
- Schreier S, Triampo W. Systemic cytology. A novel diagnostic approach for assessment of early systemic disease. Med Hypotheses 2021;156:110682. [Crossref] [PubMed]
- Sobhani N, Chahwan R, Roudi R, et al. Predictive and Prognostic Value of Non-Coding RNA in Breast Cancer. Cancers (Basel) 2022;14:2952. [Crossref] [PubMed]
- Lewis BP, Burge CB, Bartel DP. Conserved seed pairing, often flanked by adenosines, indicates that thousands of human genes are microRNA targets. Cell 2005;120:15-20. [Crossref] [PubMed]
- Calin GA, Croce CM. MicroRNA signatures in human cancers. Nat Rev Cancer 2006;6:857-66. [Crossref] [PubMed]
- Richard V, Davey MG, Annuk H, et al. MicroRNAs in Molecular Classification and Pathogenesis of Breast Tumors. Cancers (Basel) 2021;13:5332. [Crossref] [PubMed]
- Arroyo JD, Chevillet JR, Kroh EM, et al. Argonaute2 complexes carry a population of circulating microRNAs independent of vesicles in human plasma. Proc Natl Acad Sci U S A 2011;108:5003-8. [Crossref] [PubMed]
- Richard V, Davey MG, Annuk H, et al. The double agents in liquid biopsy: promoter and informant biomarkers of early metastases in breast cancer. Mol Cancer 2022;21:95. [Crossref] [PubMed]
- Alunni-Fabbroni M, Majunke L, Trapp EK, et al. Whole blood microRNAs as potential biomarkers in post-operative early breast cancer patients. BMC Cancer 2018;18:141. [Crossref] [PubMed]
- Akkiprik M, Koca S, Uğurlu MÜ, et al. Response Assessment With Molecular Characterization of Circulating Tumor Cells and Plasma MicroRNA Profiling in Patients With Locally Advanced Breast Cancer During Neoadjuvant Chemotherapy. Clin Breast Cancer 2020;20:332-343.e3. [Crossref] [PubMed]
- Madhavan D, Zucknick M, Wallwiener M, et al. Circulating miRNAs as surrogate markers for circulating tumor cells and prognostic markers in metastatic breast cancer. Clin Cancer Res 2012;18:5972-82. [Crossref] [PubMed]
- Fischer C, Deutsch TM, Feisst M, et al. Circulating miR-200 family as predictive markers during systemic therapy of metastatic breast cancer. Arch Gynecol Obstet 2022;306:875-85. [Crossref] [PubMed]
- Fischer C, Turchinovich A, Feisst M, et al. Circulating miR-200 Family and CTCs in Metastatic Breast Cancer before, during, and after a New Line of Systemic Treatment. Int J Mol Sci 2022;23:9535. [Crossref] [PubMed]
- Guo W, Lu X, Liu Q, et al. Prognostic value of neutrophil-to-lymphocyte ratio and platelet-to-lymphocyte ratio for breast cancer patients: An updated meta-analysis of 17079 individuals. Cancer Med 2019;8:4135-48. [Crossref] [PubMed]
- Gianni C, Palleschi M, Schepisi G, et al. Circulating inflammatory cells in patients with metastatic breast cancer: Implications for treatment. Front Oncol 2022;12:882896. [Crossref] [PubMed]
- De Giorgi U, Mego M, Scarpi E, et al. Association between circulating tumor cells and peripheral blood monocytes in metastatic breast cancer. Ther Adv Med Oncol 2019;11:1758835919866065. [Crossref] [PubMed]
- Smietanka U, Szostakowska-Rodzos M, Tabor S, et al. Clusters, Assemblies and Aggregates of Tumor Cells in the Blood of Breast Cancer Patients; Composition, Mode of Action, Detection and Impact on Metastasis and Survival. Int J Transl Med 2021;1:55-68. [Crossref]
- Carmona-Ule N, González-Conde M, Abuín C, et al. Short-Term Ex Vivo Culture of CTCs from Advance Breast Cancer Patients: Clinical Implications. Cancers (Basel) 2021;13:2668. [Crossref] [PubMed]
- Pereira-Veiga T, Bravo S, Gómez-Tato A, et al. Red Blood Cells Protein Profile Is Modified in Breast Cancer Patients. Mol Cell Proteomics 2022;21:100435. [Crossref] [PubMed]
- In ‘t Veld SGJG, Wurdinger T. Tumor-educated platelets. Blood 2019;133:2359-64. [Crossref] [PubMed]
- Best MG, Sol N, Kooi I, et al. RNA-Seq of Tumor-Educated Platelets Enables Blood-Based Pan-Cancer, Multiclass, and Molecular Pathway Cancer Diagnostics. Cancer Cell 2015;28:666-76. [Crossref] [PubMed]
- Mego M, Gao H, Cohen EN, et al. Circulating Tumor Cells (CTC) Are Associated with Defects in Adaptive Immunity in Patients with Inflammatory Breast Cancer. J Cancer 2016;7:1095-104. [Crossref] [PubMed]
- Szczerba BM, Castro-Giner F, Vetter M, et al. Neutrophils escort circulating tumour cells to enable cell cycle progression. Nature 2019;566:553-7. [Crossref] [PubMed]
- Cole S, Montero A, Garret-Mayer E, et al. Elevated Circulating Myeloid Derived Suppressor Cells (MDSC) Are Associated with Inferior Overall Survival (OS) and Correlate with Circulating Tumor Cells (CTC) in Patients with Metastatic Breast Cancer. Cancer Res 2009;69:4135. [Crossref]
- Bergenfelz C, Roxå A, Mehmeti M, et al. Clinical relevance of systemic monocytic-MDSCs in patients with metastatic breast cancer. Cancer Immunol Immunother 2020;69:435-48. [Crossref] [PubMed]
- Bidard FC, Mathiot C, Degeorges A, et al. Clinical value of circulating endothelial cells and circulating tumor cells in metastatic breast cancer patients treated first line with bevacizumab and chemotherapy. Ann Oncol 2010;21:1765-71. [Crossref] [PubMed]
- Codes M, Manso L, Ciruelos EM, et al. Value of circulating epithelial tumor cells and circulating endothelial cells (CTCs/CECs) in patients with HER2-negative recurrent or metastatic breast cancer treated with bevacizumab (B) in combination with paclitaxel (P) and gemcitabine (G) as first-line therapy (AVALUZ trial). J Clin Oncol 2012;30:e21088. [Crossref]
- Mäurer M, Schott D, Pizon M, et al. Increased Circulating Epithelial Tumor Cells (CETC/CTC) over the Course of Adjuvant Radiotherapy Is a Predictor of Less Favorable Outcome in Patients with Early-Stage Breast Cancer. Curr Oncol 2022;30:261-73. [Crossref] [PubMed]
- Gerber T, Taschner-Mandl S, Saloberger-Sindhöringer L, et al. Assessment of Pre-Analytical Sample Handling Conditions for Comprehensive Liquid Biopsy Analysis. J Mol Diagn 2020;22:1070-86. [Crossref] [PubMed]
- Schneegans S, Lück L, Besler K, et al. Pre-analytical factors affecting the establishment of a single tube assay for multiparameter liquid biopsy detection in melanoma patients. Mol Oncol 2020;14:1001-15. [Crossref] [PubMed]
- Keup C, Suryaprakash V, Hauch S, et al. Integrative statistical analyses of multiple liquid biopsy analytes in metastatic breast cancer. Genome Med 2021;13:85. [Crossref] [PubMed]
- Keup C, Suryaprakash V, Storbeck M, et al. Longitudinal Multi-Parametric Liquid Biopsy Approach Identifies Unique Features of Circulating Tumor Cell, Extracellular Vesicle, and Cell-Free DNA Characterization for Disease Monitoring in Metastatic Breast Cancer Patients. Cells 2021;10:212. [Crossref] [PubMed]
- Hofmann L, Sallinger K, Haudum C, et al. A Multi-Analyte Approach for Improved Sensitivity of Liquid Biopsies in Prostate Cancer. Cancers (Basel) 2020;12:2247. [Crossref] [PubMed]
- Setayesh SM, Hart O, Naghdloo A, et al. Multianalyte liquid biopsy to aid the diagnostic workup of breast cancer. NPJ Breast Cancer 2022;8:112. [Crossref] [PubMed]
- Yang Z, LaRiviere MJ, Ko J, et al. A Multianalyte Panel Consisting of Extracellular Vesicle miRNAs and mRNAs, cfDNA, and CA19-9 Shows Utility for Diagnosis and Staging of Pancreatic Ductal Adenocarcinoma. Clin Cancer Res 2020;26:3248-58. [Crossref] [PubMed]
- Keup C, Kimmig R, Kasimir-Bauer S. Combinatorial Power of cfDNA, CTCs and EVs in Oncology. Diagnostics (Basel) 2022;12:870. [Crossref] [PubMed]
Cite this article as: Nicolò E, Gianni C, Pontolillo L, Serafini MS, Munoz-Arcos LS, Andreopoulou E, Curigliano G, Reduzzi C, Cristofanilli M. Circulating tumor cells et al.: towards a comprehensive liquid biopsy approach in breast cancer. Transl Breast Cancer Res 2024;5:10.