Translating new breast ultrasound techniques into clinical practice: evaluating their intended uses and describing other unexpected uses for them
Introduction
Background
Conventional B-mode ultrasound has been used detect and differentiate breast lesions (1,2).
New ultrasound tools have been developed to further evaluate breast lesions. These include strain elastography (SRE), MicroPure Imaging (MP) and superb microvascular imaging (SMI).
Rationale and knowledge gap
These techniques have had varying success in their translation into clinical practice. SRE findings was not previously incorporated into the American College of Radiology (ACR) Breast Imaging Reporting and Data System (BI-RADS) atlas and lexicon but was included in the latest 2013 5th edition. Although calcifications and vascularity are included in the BI-RADS ultrasound assessment, specific use of MP and SMI and examples of their use are not in the latest edition of the atlas.
Objective
This article describes the intended uses of these applications, reviews the studies evaluating them and discusses some uses of these tools for which they were not originally intended.
Three newer ultrasound tools
SRE
Ultrasound elastography is a technique that provides information on tissue elasticity and stiffness. It is based on the fact that tissue elasticity can be a predictor of malignancy with benign lesions being more elastic and malignant lesions being stiffer. SRE is a semiquantitative technique with displacement of the tissue from manual compression with the ultrasound transducer or from the patient’s cardiac and respiratory movements. The results may be reported as a score based on a colour map scheme, or as a strain ratio (SR) or fat-lesion ratio (FLR) (Figure 1).
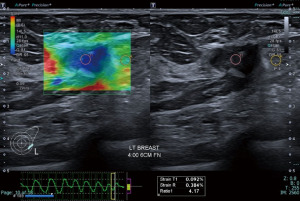
A meta-analysis (3) in 2012 of nine studies of the accuracy of quantitative ultrasound elastography for differentiation of malignant and benign breast abnormalities showed overall sensitivity and specificity of 88% but found a wide range of cut-off values from 0.5 to 4.5.
The World Federation for Ultrasound in Medicine and Biology (WFUMB) published guidelines in 2015 for breast elastography (4,5). These guidelines acknowledged also the marked variance in SR cut off values used in differentiating benign from malignant lesions.
A subsequent meta-analysis (6) in 2022 reviewed 65 studies. This found that despite the WFUMB guidelines, breast SR measurement was still laden with multiple heterogeneities. These lie within the whole range of assessments involving the patient selection, index test, reference standard and flow and timing.
In discussion of the possibility that manufacturer models may influence SR values, the review found that most of the same model subgroups did not reproduce the same favourable threshold effects and having a machine predetermined cut-off value at this stage would require more robust evidence than currently available. The review also concluded that currently the optimal breast SR cut-off point or value remains unresolved.
One common finding on elastography is the artefact seen with breast cysts. These have two characteristic appearances (7,8), the bullseye artefact or the blue-green-red (BGR) trilaminar artefact with blue being the superficial colour and red the deep one (Figure 2).
While most simple cysts show typical sonographic features of circumscribed margins, anechoic content with posterior acoustic shadowing, there are instances where inspissated contents may make the diagnosis less straightforward. SRE may be useful in these circumstances. SRE may also be useful in demonstrating the cystic component of a complex solid-cystic lesion. This may help target the solid component for histological correlation (Figures 3,4).
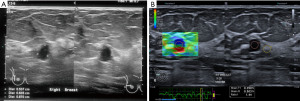
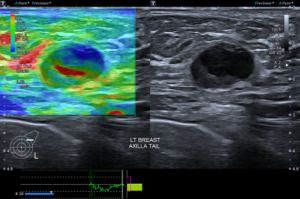
SMI
Doppler ultrasound (including colour and power Doppler) has been used to evaluate the internal vascularity in solid breast lesions. They supplement B-mode imaging by additionally demonstrating features suggestive of malignancy including the presence of both peripheral and central vascularity, penetrating vessels, and branching vessels (9-11).
However, these Doppler technologies remove clutter by suppressing low velocity components. This suppression results in a loss of data, and subsequent loss of visibility of flow in smaller vessels <0.1 mm in diameter including micro-vessels in breast cancer (12). This loss of Doppler signals from intra-tumoral micro-vessels in breast cancer may result in poorer correlation between the vascular features seen on ultrasound and micro-vessel density on pathological examination (12,13).
Instead of suppressing these low flow signals, SMI analyses clutter motion and uses an adaptive algorithm to identify and separate these flow signals from overlaying tissue motion artifacts. This results in a high resolution ultrasound image in which minute vessels and low velocity flow can be demonstrated. All this can also be done at high frame rates. As a result, SMI can provide more detailed microvascular information about breast tumours (Figures 5,6).
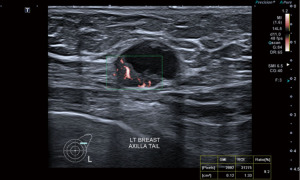
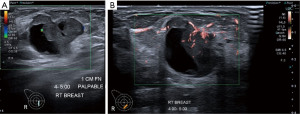
Comparative studies of the performance of B-mode versus B-mode with SMI have been published. These show improved diagnostic capability in distinguishing benign and malignant lesions (particularly in the BI-RADS 4 category) using vascular quality, morphology and distribution characteristics (14,15).
The vascular index (VI) is a parameter that is determined by SMI to quantify flow signals as the ratio of colour pixels to all pixels within the mass. Studies have shown good interobserver reproducibility of VI (16,17). There is also improved diagnostic performance in distinguishing benign from malignant lesions with malignant lesions showing a significantly higher VI. However, different cut off values (2.95–4) were determined or used in each study.
One study (18) also showed improvement in BI-RADS categorization based on VI compared to B-mode imaging.
Apart from solid masses, SMI may also demonstrate micro-vascularity within the solid component of complex solid-cystic lesions (Figure 5). This may be useful in distinguishing these complex lesions from complicated cysts with echogenic inspissated content and simple cysts with some reverberation artefact posterior to the superficial cyst wall. The vascular solid component would require histological evaluation to exclude malignancy (Figure 7A,7B).
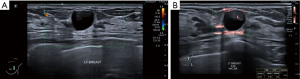
MP
MP is an ultrasound processing technique to highlight microcalcifications. This combines tissue harmonic imaging (which allows improvement of spatial resolution to aid detection) with speckle suppression using a constant false alarm rate filter. This filter suppresses visualisation of fat, mammary gland borders and chest wall musculature and reduces echogenic areas from the structures which may mimic microcalcifications. It also extracts only isolated high-brightness echoes against the heterogeneous background (19,20). By comparing the average brightness within a small area to that of the central pixel and calculating the difference, these brighter areas representing microcalcification are prominently displayed as white spots superimposed on a blue background.
A few studies have demonstrated superior detection of larger numbers of microcalcifications with MicroPure ultrasound imaging than with B-mode ultrasound (19-22).
However, using MP to detect calcifications in a breast screening setting has been deemed inappropriate. Although a study found that evaluations of the presence or absence of microcalcifications did not significantly differ from those of mammography, accuracy was low overall with mean values of 52% (23).
Another study (24) found that malignant cases were seen to have significantly more microcalcifications than benign cases and MP can be used to identify areas with breast microcalcifications but cannot effectively characterize such areas as benign or malignant.
Studies have also suggested that MP may be used to target areas with calcifications for biopsy (22,24).
Some clinical scenarios where this may be helpful would include:
Firstly, cases with mammographically indeterminate or suspicious calcifications and a hypoechoeic lesion on ultrasound with possible calcifications. MP may demonstrate calcifications in the lesion which correlate with those on mammography. This would suggest a single lesion which may be targeted on ultrasound.
Secondly, cases with calcifications on mammography but patient unable to tolerate mammographically guided biopsy. Demonstration of the calcifications on ultrasound would allow ultrasound biopsy under sedation or anaesthesia without the need for mammographic guidance or breast compression.
Thirdly, cases where calcifications on mammography are at a difficult location for mammographically guided biopsy such as in the posterior aspect of the breast or close to an implant. Ultrasound guided biopsy with MP can be more readily performed (Figure 8A-8E).
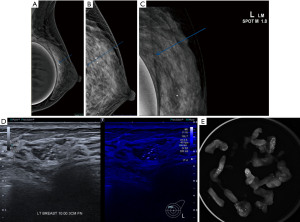
The following case is one such example. A 30-year-old female with prior breast implants is on follow up for bilateral nodules. Mammography detected a tight cluster of calcifications in the posterior superior aspect of the left breast. These could not be visualised on the craniocaudal view. There are also other calcifications within the same breast with a mammographically benign appearance. Ultrasound showed a possible correlative finding in the upper inner quadrant and MP shows this is likely to be the cluster seen on mammography. Ultrasound guided vacuum biopsy was then performed and specimen radiograph confirmed the suspicious cluster of calcifications within the cores. Histology showed a fibroadenoma and ductal carcinoma in situ.
Discussion
SRE, SMI and MP are three techniques which supplement B-mode and Doppler imaging of the breasts.
SRE has been shown to increase the sensitivity of detecting malignant lesions when added to B-mode imaging although the cut off for the SR for malignant lesions varies greatly between studies and a standardised cut off value has yet to be determined.
It is also useful in demonstrating a unique trilaminar artefact in cystic lesions as well as the cystic component of complex solid-cystic lesions.
SMI (with VI) has been shown to be helpful in distinguishing benign from malignant lesions.
It is also useful in demonstrating small vessels with slow flow in the solid component of solid-cystic lesions which should be further targeted for histological correlation. These may not be visualised on colour Doppler imaging.
MP has not been shown to be an effective replacement for mammography in screening for breast calcifications which may indicate underlying malignancy. It is also not effective in determining if a lesion is benign or malignant based on the appearance of the calcifications seen on ultrasound. However, in cases with mammographic calcifications and a sonographic lesion, demonstrating calcification within the lesion on ultrasound would increase confidence of correlating the two lesions as one. It may also provide real-time guidance for targeting these calcifications sonographically during biopsy.
Conclusions
The above scenarios illustrate the fact that in translating newly developed tools into clinical practice, there are often mixed results. Some of these tools do not fulfil the purpose for which they were initially developed. They are sadly “lost in translation” so to speak. However, in applying these tools, there may be valuable unexpected uses for which they were not initially developed. It is hoped that these may be incorporated into clinical practice guidelines and references atlases in future. These scenarios are a reminder for their users to remain open and perceptive to the potentially useful applications which may be “found in translation”.
Acknowledgments
Funding: None.
Footnote
Peer Review File: Available at https://tbcr.amegroups.com/article/view/10.21037/tbcr-23-29/prf
Conflicts of Interest: The author has completed the ICMJE uniform disclosure form (available at https://tbcr.amegroups.com/article/view/10.21037/tbcr-23-29/coif). The author has no conflicts of interest to declare.
Ethical Statement: The author is accountable for all aspects of the work in ensuring that questions related to the accuracy or integrity of any part of the work are appropriately investigated and resolved.
Open Access Statement: This is an Open Access article distributed in accordance with the Creative Commons Attribution-NonCommercial-NoDerivs 4.0 International License (CC BY-NC-ND 4.0), which permits the non-commercial replication and distribution of the article with the strict proviso that no changes or edits are made and the original work is properly cited (including links to both the formal publication through the relevant DOI and the license). See: https://creativecommons.org/licenses/by-nc-nd/4.0/.
References
- Guo R, Lu G, Qin B, et al. Ultrasound Imaging Technologies for Breast Cancer Detection and Management: A Review. Ultrasound Med Biol 2018;44:37-70. [Crossref] [PubMed]
- Hooley RJ, Scoutt LM, Philpotts LE. Breast ultrasonography: state of the art. Radiology 2013;268:642-59. [Crossref] [PubMed]
- Sadigh G, Carlos RC, Neal CH, et al. Accuracy of quantitative ultrasound elastography for differentiation of malignant and benign breast abnormalities: a meta-analysis. Breast Cancer Res Treat 2012;134:923-31. [Crossref] [PubMed]
- Shiina T, Nightingale KR, Palmeri ML, et al. WFUMB guidelines and recommendations for clinical use of ultrasound elastography: Part 1: basic principles and terminology. Ultrasound Med Biol 2015;41:1126-47. [Crossref] [PubMed]
- Barr RG, Nakashima K, Amy D, et al. WFUMB guidelines and recommendations for clinical use of ultrasound elastography: Part 2: breast. Ultrasound Med Biol 2015;41:1148-60. [Crossref] [PubMed]
- Mutala TM, Mwango GN, Aywak A, et al. Determining the elastography strain ratio cut off value for differentiating benign from malignant breast lesions: systematic review and meta-analysis. Cancer Imaging 2022;22:12. [Crossref] [PubMed]
- Dietrich CF, Barr RG, Farrokh A, et al. Strain Elastography - How To Do It? Ultrasound Int Open 2017;3:E137-49. [Crossref] [PubMed]
- Barr RG. Future of breast elastography. Ultrasonography 2019;38:93-105. [Crossref] [PubMed]
- Lee SW, Choi HY, Baek SY, et al. Role of color and power doppler imaging in differentiating between malignant and benign solid breast masses. J Clin Ultrasound 2002;30:459-64. [Crossref] [PubMed]
- Lee WJ, Chu JS, Huang CS, et al. Breast cancer vascularity: color Doppler sonography and histopathology study. Breast Cancer Res Treat 1996;37:291-8. [Crossref] [PubMed]
- Kook SH, Park HW, Lee YR, et al. Evaluation of solid breast lesions with power Doppler sonography. J Clin Ultrasound 1999;27:231-7. [Crossref] [PubMed]
- Schroeder RJ, Bostanjoglo M, Rademaker J, et al. Role of power Doppler techniques and ultrasound contrast enhancement in the differential diagnosis of focal breast lesions. Eur Radiol 2003;13:68-79. [Crossref] [PubMed]
- Buadu LD, Murakami J, Murayama S, et al. Colour Doppler sonography of breast masses: a multiparameter analysis. Clin Radiol 1997;52:917-23. [Crossref] [PubMed]
- Zhu YC, Zu DM, Zhang Y, et al. A comparative study on superb microvascular imaging and conventional ultrasonography in differentiating BI-RADS 4 breast lesions. Oncol Lett 2019;18:3202-10. [Crossref] [PubMed]
- Park AY, Seo BK, Woo OH, et al. The utility of ultrasound superb microvascular imaging for evaluation of breast tumour vascularity: comparison with colour and power Doppler imaging regarding diagnostic performance. Clin Radiol 2018;73:304-11. [Crossref] [PubMed]
- Chae EY, Yoon GY, Cha JH, et al. Added Value of the Vascular Index on Superb Microvascular Imaging for the Evaluation of Breast Masses: Comparison With Grayscale Ultrasound. J Ultrasound Med 2021;40:715-23. [Crossref] [PubMed]
- Lee EJ, Chang YW, Oh E, et al. Reproducibility and diagnostic performance of the vascular index of superb microvascular imaging in real-time breast ultrasonography for evaluating breast masses. Ultrasonography 2021;40:398-406. [Crossref] [PubMed]
- Cai SM, Wang HY, Zhang XY, et al. The Vascular Index of Superb Microvascular Imaging Can Improve the Diagnostic Accuracy for Breast Imaging Reporting and Data System Category 4 Breast Lesions. Cancer Manag Res 2020;12:1819-26. [Crossref] [PubMed]
- Park AY, Seo BK, Cho KR, et al. The Utility of MicroPure™ Ultrasound Technique in Assessing Grouped Microcalcifications without a Mass on Mammography. J Breast Cancer 2016;19:83-6. [Crossref] [PubMed]
- Machado P, Eisenbrey JR, Stanczak M, et al. Ultrasound Detection of Microcalcifications in Surgical Breast Specimens. Ultrasound Med Biol 2018;44:1286-90. [Crossref] [PubMed]
- Machado P, Eisenbrey JR, Cavanaugh B, et al. New image processing technique for evaluating breast microcalcifications: a comparative study. J Ultrasound Med 2012;31:885-93. [Crossref] [PubMed]
- Tan R, Xiao Y, Tang Q, et al. The Diagnostic Value of Micropure Imaging in Breast Suspicious Microcalcificaion. Acad Radiol 2015;22:1338-43. [Crossref] [PubMed]
- Machado P, Eisenbrey JR, Cavanaugh B, et al. Microcalcifications versus artifacts: initial evaluation of a new ultrasound image processing technique to identify breast microcalcifications in a screening population. Ultrasound Med Biol 2014;40:2321-4. [Crossref] [PubMed]
- Machado P, Eisenbrey JR, Stanczak M, et al. Characterization of Breast Microcalcifications Using a New Ultrasound Image-Processing Technique. J Ultrasound Med 2019;38:1733-8. [Crossref] [PubMed]
Cite this article as: Ong EMW. Translating new breast ultrasound techniques into clinical practice: evaluating their intended uses and describing other unexpected uses for them. Transl Breast Cancer Res 2023;4:23.