Features and applications of Ehrlich tumor model in cancer studies: a literature review
Introduction
In 2020, breast cancer became the most common cancer worldwide, surpassing lung cancer. Among women, breast cancer is the leading cause of cancer deaths. In the same year, more than 2.26 million new cases of breast cancer and nearly 685,000 deaths from breast cancer occurred worldwide (1). With these high numbers, breast cancer has enormous social and familial impacts. One notable impact is the creation of orphans that is attributed to maternal cancer mortality. There were 1,047,000 new orphans in 2020, and 25% (258,000)of these were attributable to maternal deaths from breast cancer. These children experience health and education disadvantages throughout their lives (2).
Breast cancer also affects health systems in many countries by having high treatment costs, especially in advanced stages of the disease (3,4). Breast cancer is among the top five cancers with the highest economic costs, together with tracheal, bronchus, and lung cancer; colon and rectum cancer; liver cancer; and leukemia (1). Costs that are associated with breast cancer in the United States increased from $26.8 to $29.8 billion from 2015 to 2020 (5). A substantial proportion of these costs are associated with breast cancer treatment, which is based on the tumor subtype and stage of progression, with or without metastasis.
According to specific subtypes, breast cancer is classified based on the expression of estrogen receptors (ERs), progesterone receptors (PRs), and human epidermal growth factor 2 (HER2): luminal A (HER2−), luminal B (HER2+/−), HER2 overexpression (ER−/PR−/HER2+), and triple-negative (ER−/PR−/HER2−), the latter of which is the most severe (6). The importance of ERα in the origin of breast cancer was recently suggested based on the induction of dicentric chromosome bridge formation and breakage that cause the amplification of key oncogenes, including ERBB2/HER2 and cyclin D1 (CCND1), in breast cancer cells (7).
Therapies for breast cancer are based on the cancer subtype and include surgery, chemotherapy, radiotherapy, and immunotherapy. Numerous anticancer drugs are currently available, but these treatments have low efficacy and high toxicity, leading to cancer progression, cancer recurrence, and low survival (8). New safe and effective therapies with low toxicity and a lower cost are still needed. In vitro and in vivo models are important tools for pharmacological investigations. Ehrlich tumors in mice are one of these models, used for more than a century. Ehrlich cells (ECs) have characteristics that are very close to human breast cancer cells, representing a hormone-positive tumor model. The present review discusses the cellular, biochemical, and genetic characteristics of the Ehrlich tumor model, its applications in pharmacological, pathological, and translational studies, and laboratory research manipulations of Ehrlich tumor cells. We present this article in accordance with the Narrative Review reporting checklist (available at https://tbcr.amegroups.com/article/view/10.21037/tbcr-23-32/rc).
Methods
This narrative review was based on scientific articles on Ehrlich tumors that have been published by scientists from several countries. The literature search was performed in the PubMed, SciELO, Google Scholar, Google, and Clarivate (Web of Science) databases. Table 1 presents the search strategy.
Table 1
Items | Specification |
---|---|
Date of search | February to June 2023 |
Databases and sources searched | PubMed, SciELO, Google Scholar, Google, Clarivate, including thesis and books |
Search terms | “Ehrlich tumor”; “Ehrlich tumor” and “breast cancer”, “metabolism”, “treatment”, “translational”, “features”, “immunological”, “cancer”, “oxidative stress”, “protein expression”, “immune response”, “cachexia” |
Timeframe | 1947–2023 |
Inclusion and exclusion criteria | Inclusion: articles, books, and thesis with recognizable authors and citations. Exclusion: articles with only abstract available; sources published in languages others than English, Portuguese, or Spanish |
Selection process | All authors selected and shared with each other the published literature sources |
Historical approach of Ehrlich tumors
Ehrlich tumors are a model that is largely used in cancer research, both solid and ascitic forms (9). This tumor is considered a carcinoma. By definition, this means a cancer that arises in epithelial tissue of the skin or lining of internal organs. The Ehrlich tumor originated in breast tissue. It is a spontaneous mammary adenocarcinoma in female mice that was first transplanted by its namesake Paul Ehrlich. Ehrlich and Apolant [1905] used an Ehrlich tumor in an experiment by transplanting tumor tissues subcutaneously from mouse to mouse. The first experiments with Ehrlich tumors were performed with solid Ehrlich carcinoma (SEC). Later, in 1932, the tumor cells began to be manipulated as ascitic Ehrlich carcinoma (AEC). After 1940, studies began to standardize both types of Ehrlich tumors, adapting them for qualitative and quantitative research (10). Since then, AEC cells have spread rapidly among research institutes globally (11).
Since the establishment of Ehrlich tumors, they have been applied to studies of the experimental oncology, biochemistry, pharmacology, and carcinogenesis of breast cancer. Since 1948, approximately 14,500 articles that mentioned this tumor have been published. According to PubMed [2023], the highest scientific literature production with Ehrlich tumors was reached in the 1960s, with a decrease after the 1990s. From 2010 until now, published articles that mention Ehrlich tumors have again increased. This tumor has been used to investigate systemic alterations that are induced by tumors, the sensitivity of tumor cells to chemotherapies, and the antitumor potential of synthetic and natural products.
Ehrlich tumor types and characteristics
ECs can produce ascitic or solid tumors, depending on the site of inoculation. AEC originates from intraperitoneal inoculation, where tumor cells may lead to an increase in peritoneal vascular permeability, generating an aggressivity tumor form (10,11). Macroscopically, ascitic fluid (Figure 1A,1B) is viscous and colored light gray or beige. Over time, it may develop a reddish color as a result of intraperitoneal hemorrhage. The death of the animal with AEC occurs between 10 and 14 days after cell inoculation, depending on different situations, such as (I) the concentration of inoculated cells, (II) the amount of fluid in the peritoneal cavity, which causes abdominal pressure and compression of the organs, and (III) the number of passages, in which repeated transplantation increases malignancy and tumor proliferation (10). Intraperitoneal passages gradually increase the cell proliferation rate, resulting in an ascitic tumor that resembles human tumors that are sensitive to chemotherapy (11).
In contrast, SEC is obtained by subcutaneous inoculation in the dorsal region (Figure 1C), paws (Figure 1D), or upper region of the head (12-19), inducing a solid tumor 14 days after inoculation (20,21), which is palpable from day 8 (16,18) and can be maintained by day 42 after inoculation (21). Tumor growth can be observed exponentially (Figure 1E) on a daily basis, in which the tumor mass is fibrous, firm, and well-defined (10). This model has been frequently used in pharmacological studies, considering the longest survival time (compared to other models), high transplantable capability, fast and undifferentiated growth, no regression, and low malignancy (9,11,21).

SEC tumor cells are anaplastic and pleomorphic and have a high nucleus/cytoplasm ratio. The nucleus is composed of nucleoli and loose chromatin, and a high mitotic level can be identified. The stromal constitution comprises delicate capillaries and collagen fibers. It is also possible to identify areas of necrosis and hemorrhage. These cells still express anti-proliferating cell nuclear antigen (PCNA) with nuclear labeling, indicating lower positivity at day 7 than at day 14. AEC exhibits histological features that are similar to SEC but with larger cells. However, anti-PCNA staining was higher in the 7-day group than in the 14-day group, indicating that the difference in PCNA expression is related to a difference in the growth curve between the ascitic and solid forms (22).
Histologically, SEC is composed of neoplastic cells, connective tissue, and polymorphonuclear lymphocytes, which are all part of a well-defined capsule. Cellular composition is heterogeneous in different zones of SEC, such as in central areas relative to the periphery, with inflammatory cells near a necrotic zone. The periphery of the tumor is characterized by neoplastic cells, frequently in mitosis, and large heteroplasmy with polymorphism in both cells and the nucleus. Other areas are composed of cells with different phenotypes in acinus-like structures (9).
Evaluations of SEC have shown that the tumor has a capsule that is detectable by Doppler ultrasound, although this is not present in all cases, and it also exhibits blood flow, which may be reduced to central or distinct foci of necrosis. Tumor development varies according to its vascularization. Greater vascularization is associated with a faster growth rate. Conversely, the absence of blood flow and a homogeneous echotexture are associated with a slower growth rate of tumor cells. Additionally, extratumor vessels have a significantly higher resistivity index (RI) and pulsatility index (PI) than tumor vessels (23). Treatment with polysaccharides from sweet green pepper was shown to reduce vascular endothelial growth factor (Vegf) gene expression and vessel diameter but not the number of vessels in SEC in mice that were treated orally for 21 days. This effect was related to a reduction of tumor growth in these animals (18), confirming that vascularization is a key factor for SEC development.
EC preparation and manipulation
ECs can be used both in vivo and in vitro. Regardless of the way they are eventually used, these cells must undergo an obligatory in vivo phase (i.e., serial intraperitoneal passages). The intraperitoneal passage process guarantees a degree of malignancy that is similar to the parental tumor, which can be explanted from a mouse and re-inoculated in another mouse or cultured in vitro (24). Frozen cells are maintained in a culture medium, such as Eagle, supplemented with 10% fetal bovine serum (FBS) (25), or in a solution of FBS and 10% dimethylsulfoxide (DMSO), and kept in a −80 ℃ freezer or liquid nitrogen vials.
The intraperitoneal passage period is approximately 6–10 days (16,26). Afterward, animals with AEC are euthanized, and 1 ml of sterile phosphate-buffered saline (PBS) is injected in the peritoneal cavity to dilute the liquid tumor, followed by a small incision of the peritoneum to allow ascitic fluid collection under aseptic conditions. The cells are then washed, centrifuged at 4 ℃, and resuspended to measure cell viability (26,27). Cell viability is usually evaluated by trypan blue staining and must be above 95% to be considered satisfactory for application in experiments and SEC formation; otherwise, the process of intraperitoneal passages is continued (26). The washing and resuspension of Ehrlich carcinoma cells are generally performed using isotonic solutions, such as PBS and sodium chloride (NaCl). Schachtschabel & Foley (25) reported that although Ehrlich carcinoma cells are tolerant cells, in a hypertonic environment they abandon their epithelial morphology to become similar to fibroblasts, with an extended appearance, in addition to an increasing cell duplication time.
The subsequent standard cell dose that is used is based on the number of cells that are needed to generate a lethal ascitic tumor in 100% of inoculated mice, which is 106 viable cells. Under these conditions, animals develop an ascitic tumor and die within 30 days (24). On day 8 of tumor growth, there are 140–280 million cells/mL of ascitic fluid, in a total volume of 5–8 mL (24). Most often, a higher number of inoculated cells results in a shorter duration of intraperitoneal passage (28). The recommended intraperitoneal inoculation volume is below 0.2 mL, sometimes without specifying the exact number of cells (27,29). Figure 2 shows the process of EC manipulation for the development of SEC and AEC.
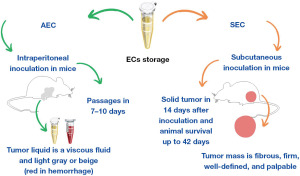
Immune and systemic alterations caused by Ehrlich tumors
Immunoinflammatory regulation
Differences in the inoculation and constitution of AEC and SEC tumors are marked by differences in the immune response, metabolism, and other pathophysiological aspects. Some studies reported relevant immune responses against these cells, but others reported escape strategies of tumors against the immune system. Most studies describe AEC as responsible for promoting higher immune modulations compared with SEC (30).
Tumor cell proliferation is marked by an immune modulation response, in which immune cells interact with tumor cells in the tumor microenvironment. Ozaslan et al. reported that AEC has a higher capacity for proliferation and immune evasion because it does not have a specific tumor transplant antigen (11). Instead, in AEC, splenic cells are altered, such as a decrease in CD4 and CD8 T cells, an increase in B-cell number (which peaked 2 days after tumor inoculation), and an increase in the macrophage population (31). Inzhevatkin et al. reported the suppression of metabolic processes of macrophages that were associated with tumors during tumor growth. Neutrophils are another important cell population in tumor development (32). AEC plays a paradoxical dual role in these cells, in which neutrophils are responsible for controlling tumor development in susceptible animals (e.g., Swiss mice), but in mice that are resistant to tumor development [e.g., CAF1 (BALB/c A/J) F1 mice], neutrophils improve tumor growth (33).
Additionally, thymic damage occurs at late stages of cancer, specifically at day 21 after tumor inoculation, marked by the depletion of CD4+ and CD8+ cells in peripheral blood (34). All actions of immune cells in the tumor microenvironment result in cytokine expression, especially proinflammatory cytokines [e.g., interleukin-1 (IL-1), IL-4, IL-6, IL-17, and tumor necrosis factor α], the enzymes myeloperoxidase and N-acetylglucosaminidase, nitric oxide, and the anti-inflammatory cytokine IL-10 (17,18,35). IL-17 is stimulated in CD4+ cells by suppressor cells that derive from myeloid-derived suppressor cells and tumor-associated macrophages (35). IL-6 is mainly involved in cachexia and metabolic changes (36). IL-1β increases on day 10 after inoculation but declines after this period, whereas interferon γ (IFN-γ) progressively increases afterward (37). Therefore, AEC is marked by a systemic inflammatory process, with neutrophilia, an increase in macrophages, thrombocytopenia, splenomegaly, and splenic hematopoiesis (31,38-40).
Peritumor inflammatory infiltrates in SEC are composed of mononuclear and polymorphonuclear leukocytes, with moderate intensity between days 2 and 7 post-inoculation. Da Silva et al. also showed a positive correlation between the intensity of inflammatory infiltrate and animal weight and tumor size (41). The main lymphocytes are CD3+, CD4+, CD8+, and Foxp3. Increases in CD3+ and Foxp3 and decreases in CD4+ and CD8+ were linked with tumor development (41). Additionally, SEC cells that were inoculated in the intraplantar area in mice were characterized by local neutrophilic infiltration with an extensive necrotic area, which may be responsible for mechanical allodynia, thermal hyperalgesia, and paw edema (42).
Metabolic changes
SEC can induce metabolic dysregulation, with a systemic proinflammatory state, skeletal muscle wasting, lipolysis, and increases in lactate dehydrogenase, creatine kinase (9), and aspartate aminotransferase (16-19). Cancer cachexia involves metabolic regulation in skeletal muscle after SEC cell implantation, which is linked with glucose oxidation, and changes in energy homeostasis and in insulin metabolism (43). Insulin secretion and glucose homeostasis are normally affected by tumor cells that consume high amounts of glucose. SEC-bearing animals exhibited decreases in glycemia, hepatic glycogen, body weight, insulinemia, and insulin sensitivity 14 days after tumor cell inoculation, associated with increases in plasma free fatty acids and triglycerides (43). From our experience, SEC can reduce hepatic glycogen without affecting glycemia and reduces the total activity of hepatic cytochrome P (8,44). Another study found that SEC also modifies fatty acid composition 23 days after tumor inoculation (45).
AEC was also associated with lower peroxidation compared with liver tissue, based on the thiobarbituric acid reactive substances method (46). The authors suggested that the resistance to lipid peroxidation is linked to lower levels of lipids and fatty acids and an increase in α-tocopherol per milligram lipid in AEC compared with the liver. However, in our experience with measuring levels of lipoperoxidation in SEC by the ferric-xylenol orange (FOX) method (47), the lipoperoxidation ratio can be higher in Ehrlich tumor tissue (4.9–10.0 nmol hydroperoxides/min/mg of protein) than in liver homogenate (2.6–8.0 nmol hydroperoxides/min/mg of protein; (8,16-19,44). Additionally, mitochondria in ascitic ECs are also resistant to lipid peroxidation mainly through the action of succinate and a glutathione-dependent mechanism (48).
Protein expression
ECs express different proteins with oncogenic characteristics that promote a series of metabolic and morphological transitions in tumor cells. These modifications are linked to structural deterioration and decreases in adenosine triphosphate concentrations and RNA and DNA synthesis (10).
Among the expressed proteins are S100A6 and CacyBP/SIP (calcyclin-binding protein and siah-1 interacting protein), which are purified in ECs and play an important role in carcinogenesis. S100A6 belongs to the S100 protein family. It is a low-molecular-weight calcium-binding protein that is also found in epithelial cells, fibroblasts, and tumor cells with high metastatic activity. CacyBP/SIP protein was discovered during analyses of S100A6. It interacts with seven in absentia homolog 1 (Siah-1) and suppressor of kinetochore protein 1 (Skp1), which are involved in the ubiquitination and degradation of β-catenin (49). In addition to tumor proteins, animals with Ehrlich tumors produce serum factors, specifically serpins, which are essential for tumor growth, tumor invasion, and apoptosis, causing an increase in the cell mitotic index from 15% to 80%. Serpins are also involved in processes of coagulation, fibrinolysis, and inflammation (50,51). Importantly, when this protein is inhibited, Ehrlich tumor-bearing animals exhibit an increase in survival from 14–20 days to 90 days (51).
Importantly, the aggressivity and resistance of AECs are linked with overexpression of the chaperone heat shock protein 70 (HSP70), which is responsible for cell proliferation, invasion, metastasis, and death. This principally occurs as an adaptive modulation of stationary ECs under conditions of chronic oxygen and nutrient deficiency (20,52). Another protein that is modulated in AEC is multidrug resistance-associated protein 1 (Mrp1), originally discovered as a cause of multidrug resistance in tumor cells. In this case, Mrp1 increased when AEC was exposed to fractional irradiation (53). Consequently, the cells can adapt and modulate their expression, increasing cell survival. Inducible nitric oxide synthase (iNOS) and cyclooxygenase 1 (COX1) protein expression in AEC increased on day 2, with peak levels at day 10 post-inoculation (37).
Ehrlich-bearing mouse welfare and physiological changes
Animal welfare and adequate animal handling are indispensable for obtaining reliable research results. Physiological changes can impair the application of tumor models to pharmacological and pathological studies. One example is immunological changes that are observed in the cohabitation of healthy and tumor-bearing mice. Maragno-Correa et al. reported a decrease in the frequency of CD4+, CD8+, and CD19+ in spleen cells in ascitic tumor-bearing animals (54). Cohabitation experiments involved pairs of animals, in which one animal was inoculated intraperitoneally with Ehrlich tumor cells, and the other was named the companion of a sick partner (CSP) that received no stimulation. For the control group, one of the animals was treated with 0.9% NaCl intraperitoneally, and the other was named the companion of a healthy partner (CHP) (55-57). These studies demonstrated numerous neurological, endocrine, and immunological changes in CSP animals. In addition to these changes, an increase in tumor growth was observed when CSP animals were inoculated with tumor cells (56). Differences were found between males and females, demonstrating different strategies in fighting the tumor. Male animals in the study by Machado et al. exhibited an increase in catecholamines and decreases in phagocytosis and oxidative burst (56). Morgulis et al. found that females exhibited increases in stress-related behavior, serum corticoid levels, and tumor growth (57). Additionally, CSP animals, when immunized with an ovalbumin booster, exhibited a series of immunological changes, such as decreases in bone marrow cell counts and plasma levels of IL-10 and IFN-γ, associated with increases in eosinophil and neutrophil counts, IL-4, IL-5, and plasma levels of norepinephrine (55). The authors indicated that the stress of cohabitation with a sick animal caused physiological and behavioral changes that increased susceptibility to Ehrlich tumor development (56).
Research applications of Ehrlich tumor model
Tumor models in preclinical studies
ECs represent a multifaceted preclinical tumor model that is applied in both pathophysiological and pharmacological screening studies for cancers. This is not limited to classic neoplasms (i.e., liquid or solid) it also extends to systemic involvement, generating such conditions as hepatotoxicity and higher levels of neoplastic markers, such as α-fetoprotein and tumor carcinoembryonic antigen, which are often observed in oncology practice (58,59). In addition to this versatility, the advantages of the model include fast tumor growth and high rates of tumor occurrence after transplantation (60). The antineoplastic potential of a wide variety of substances has already been tested, including probiotics, polysaccharides, oligosaccharides, spices, plant extracts, isolated compounds, seaweed, off-label drugs alone or in combination with routine anticancer drugs, and countless variations of formulations, including nanoparticles (8,16-19,61-65). Table 2 shows some applications of solid and liquid models of Ehrlich carcinoma in preclinical studies.
Table 2
Tumor type | Applications | References |
---|---|---|
Solid and liquid tumor | Anticancer potential of probiotics, spices, plant specimens, seaweed, and nanoparticles, alone or in combination with routine anticancer drugs | (8,19,61-66) |
Solid tumor | Chemopreventive effects of plant extracts, polysaccharides, and oligosaccharides | (8,16,18) |
Solid tumor | Antitumor/therapeutic effects of plant extracts, polysaccharides, oligosaccharides, and chemical compounds | (8,16,18,44,66-69) |
Solid and liquid tumor | Anticancer immunization | (60) |
Solid tumor | Neoplastic cachexia | (9) |
Solid tumor | Cardiac dysfunction caused by neoplastic cachexia | (27) |
Solid tumor | Pain model in cancer, indicated by mechanical and thermal hyperalgesia | (70) |
Solid tumor | Translational model of immune response | (30) |
In addition to studies of chemoprevention (71), another important point is the possibility of anticancer immunization, which can be investigated in mice that are challenged with AEC and SEC (60). These effects were more pronounced when the challenge was minimal (1,000 ECs), whereas at a medium challenge (100,000 ECs), the effect was virtually lost. Despite the translational limitation in this case, because it is a transplantable neoplasm (as opposed to what occurs in humans with an intrinsic malignant transformation), these results reinforce the importance of the immune system in fighting cancer and how animal models can broaden our knowledge. Efforts have been made to understand immune responses that are induced by ECs (30). Although coming from a similar origin, there is a distinct profile of the body’s response to ascitic and solid tumors (see section Immunoinflammatory regulation).
The Ehrlich tumor model is still important for clarifying controversial scientific issues, serving as links between in vitro and in vivo findings. One interesting example was provided by Mostafa et al., who found promising results in the application of mesenchymal stem cells in cancer cell culture, an effect that was opposite to observations in SEC under conditions of both local and systemic treatment. Mesenchymal stem cells, although presenting anti-inflammatory and regenerative effects, are affected by the tumor microenvironment, which directs them to a tumorigenic pathway only in the in vivo environment (72).
Furthermore, anticancer studies are important in terms of improving the quality of life of cancer patients. SEC triggers catabolic activities that are similar to patients with neoplastic cachexia (9). This model becomes interesting because it reproduces systemic proinflammatory characteristics, the loss of skeletal muscle, and lipolysis that are seen in human cancer patients (73). The aggressiveness of this condition is usually an experimental limiting factor, but in this model, it was possible to evaluate animals for 28 days without complications. Thus, effects of drugs on tumor growth and improvements in paraneoplastic metabolic syndromes can be studied simultaneously. Another important finding with regard to neoplastic cachexia concerns cardiac dysfunction. In this field, 24-day SEC altered cardiac structure and function, including a reduction of left ventricular wall thickness and ejection fraction, and caused degeneration, death, and muscle atrophy in the hearts of tumor-bearing mice (27). The pathogenesis of these changes is based on the suppression of mammalian/mechanistic target of rapamycin and consequent activation of autophagy. Cardiac dysfunction, in addition to being a consequence of cancer itself, is even more often caused by anticancer treatment (74).
The presence of SEC is also associated with mechanical and thermal hyperalgesia, as seen in human patients with breast cancer in the preoperative period (42). In this pain model, ECs are inoculated into the subcutaneous region of the hind paw in mice (Figure 1D), leading to local tumor development and the activation of dorsal root ganglia neurons via transient receptor potential vanilloid 1 (70). This pain-like clinical condition is responsive to the opioid morphine, which does not interfere with the tumor growth rate, so it can be used as an independent in vivo model of oncological pain.
Tumor animal models in translational studies
Translational research is complex and conducted over a long period of time. Researchers need to verify results precisely, while shortening the period between the discovery of innovations (75). The complexity of translation between tumor models occurs mainly according to the diversity of these models, which are not directly comparable. However, the models are descriptive, generally emphasizing the initial response to tumor survival of the animal and addressing tumor growth characteristics, drug effects, and in some cases resistance (76,77).
AEC is an allograft transplantation model that originates from a mouse mammary adenocarcinoma. Considering this characteristic, which is associated with rapid growth in suspension and sensitivity to chemotherapies, it can be used in translational research for breast cancer (78). SEC presents immune responses that are analogous to human breast cancers, representing a translational response, mainly to the hormone-positive breast cancer (30). Additionally, the 4T1, EMT6, TM40, and D2A1 cell lines are also considered allograft transplant models, whereas xenographic transplants include such human breast cancer cell lines as BT20, BT474, MCF-7, MDA-MB-231, and MDA-MD-453. Other animal models that are used in translational research include chemical models (e.g., 7,12-dimethylbenz[a]anthracene and N-methyl-nitrosourea), physical models that utilize radiation, biological models that utilize lentivirus infection, and genetic models that can be transgenic (e.g., oncogene activation) or knockout (e.g., through the inactivation of tumor suppressor pathways) (78).
Thus, in vitro and ex vivo models are fundamental to understanding tumor biology, identifying specific biomarkers, and searching for promising therapeutic pathways. This allows the generation of clinical results in oncology and clarification of pathways that are observed in clinical practice. However, the adopted models need to mimic the human condition, which is a challenge (79).
Limitations and conclusions
Despite several applications and advantages of the Ehrlich tumor model, such as strong reproducibility, low cost, and easy maintenance, this model has some limitations. Metastases are responsible for 90% of cancer deaths (80). ECs are not able to exhibit the invasive phenotype to produce metastasis, regardless of the route of administration of tumor cells. To analyze mechanisms that are related to metastasis in breast tumors, other models are more useful, such as those that generate lung metastases spontaneously with the inoculation of MCF-7 cells in the intra-iliac artery in mice. More recently, genetically engineered mouse models have been developed that are capable of producing spontaneous metastases in the lungs and lymph nodes (81). Another limitation of the Ehrlich model is the inability to accurately mimic defense responses to the tumor in humans. This is mainly attributable to the loss of histocompatibility complex antigens in its ascitic form (82). This is believed to be the reason for its ability to proliferate in almost all mouse lines, such Swiss and C57BL/6, despite the fact that most studies use female Swiss mice.
New in vitro, in vivo, and ex vivo models targeting different systems are necessary, which can complement existing advantages and overcome distinct disadvantages. Among these, tumor-derived organoids and organotypic cultures from fresh tumor tissue are examples of sophisticated systems (79). However, these systems also have limitations. Some of them cannot be propagated, or they cannot be easily preserved. Thus, Ehrlich tumors are a relevant tool for understanding breast cancer pathogenesis, investigating the tumor microenvironment, systemic alterations that are produced by cancer, the side effects of therapies, and discovering new treatments. Both SEC and AEC are important in preclinical and translational studies, mainly involving hormone-positive breast cancer. Indirectly, these models help to understand and minimize the problems faced in therapies and social issues related to the breast cancer, such as the impact of the disease on patients’ family.
Acknowledgments
The authors thank Dr. Claudia Rita Corso for providing the Ehrlich tumor images.
Funding: This work was supported by CNPq (No. 310290/2021-0 and No. 151344/2022-1) and CAPES (financial code 001).
Footnote
Reporting Checklist: The authors have completed the Narrative Review reporting checklist. Available at https://tbcr.amegroups.com/article/view/10.21037/tbcr-23-32/rc
Peer Review File: Available at https://tbcr.amegroups.com/article/view/10.21037/tbcr-23-32/prf
Conflicts of Interest: All authors have completed the ICMJE uniform disclosure form (available at https://tbcr.amegroups.com/article/view/10.21037/tbcr-23-32/coif). DRR was supported by CAPES (financial code 001). MCS was supported by CNPq (No. 151344/2022-1). AA was supported by CNPq (No. 310290/2021-0 and No. 151344/2022-1) and CAPES (financial code 001). The other author has no conflicts of interest to declare.
Ethical Statement: The authors are accountable for all aspects of the work in ensuring that questions related to the accuracy or integrity of any part of the work are appropriately investigated and resolved.
Open Access Statement: This is an Open Access article distributed in accordance with the Creative Commons Attribution-NonCommercial-NoDerivs 4.0 International License (CC BY-NC-ND 4.0), which permits the non-commercial replication and distribution of the article with the strict proviso that no changes or edits are made and the original work is properly cited (including links to both the formal publication through the relevant DOI and the license). See: https://creativecommons.org/licenses/by-nc-nd/4.0/.
References
- World Health Organization. Cancer. Available online: https://www.who.int/news-room/fact-sheets/detail/cancer
- Guida F, Kidman R, Ferlay J, et al. Global and regional estimates of orphans attributed to maternal cancer mortality in 2020. Nat Med 2022;28:2563-72. [Crossref] [PubMed]
- Mousa R, Hammad E, Melhem J, et al. Direct medical costs of breast cancer in Jordan: cost drivers and predictors. Expert Rev Pharmacoecon Outcomes Res 2021;21:647-54. [Crossref] [PubMed]
- Khan SA, Hernandez-Villafuerte K, Hernandez D, et al. Estimation of the stage-wise costs of breast cancer in Germany using a modeling approach. Front Public Health 2022;10:946544. [Crossref] [PubMed]
- NIH. National Cancer Institute. Financial Burden of Cancer Care 2022. Available online: https://progressreport.cancer.gov/after/economic_burden
- Johansson ALV, Trewin CB, Fredriksson I, et al. In modern times, how important are breast cancer stage, grade and receptor subtype for survival: a population-based cohort study. Breast Cancer Res 2021;23:17. [Crossref] [PubMed]
- Lee JJ, Jung YL, Cheong TC, et al. ERα-associated translocations underlie oncogene amplifications in breast cancer. Nature 2023;618:1024-32. [Crossref] [PubMed]
- Oliveira NMT, Dos Santos AE, Corso CR, et al. Chemical characterization and antineoplastic effect of oligosaccharides from Cabernet Franc red wine in mammary tumor model in mice. J Nutr Biochem 2023;113:109253. [Crossref] [PubMed]
- Frajacomo FT, de Souza Padilha C, Marinello PC, et al. Solid Ehrlich carcinoma reproduces functional and biological characteristics of cancer cachexia. Life Sci 2016;162:47-53. [Crossref] [PubMed]
- Freitas J, da Silva P, Domingues R. Manual Básico Oncologia Experimental - Tumor de Ehrlich. 2018. Available online: https://paginas.uepa.br/eduepa/index.php/2019/06/06/manual-basico-oncologia-experimental-tumor-de-ehrlich/
- Ozaslan M, Karagoz I, Kilic I, et al. Ehrlich Ascites Carcinoma. African J Biotechnol 2011;10:2375-8.
- Nascimento FR, Cruz GV, Pereira PV, et al. Ascitic and solid Ehrlich tumor inhibition by Chenopodium ambrosioides L. treatment. Life Sci 2006;78:2650-3. [Crossref] [PubMed]
- Pereira BG, Ligorio Fialho S, Maria de Souza C, et al. Evaluation of the effects of thalidomide-loaded biodegradable devices in solid Ehrlich tumor. Biomed Pharmacother 2013;67:129-32. [Crossref] [PubMed]
- de Fátima Pereira A, Mara da Costa V, Cristina Magalhães Santos M, et al. Evaluation of the effects of methotrexate released from polymeric implants in solid Ehrlich tumor. Biomed Pharmacother 2014;68:365-8. [Crossref] [PubMed]
- Miranda-Vilela AL, Grisolia CK, Longo JPF, et al. Oil rich in carotenoids instead of vitamins C and E as a better option to reduce doxorubicin-induced damage to normal cells of Ehrlich tumor-bearing mice: hematological, toxicological and histopathological evaluations. J Nutr Biochem 2014;25:1161-76. [Crossref] [PubMed]
- Corso CR, Stipp MC, Adami ER, et al. Salvia lachnostachys Benth has antitumor and chemopreventive effects against solid Ehrlich carcinoma. Mol Biol Rep 2019;46:4827-41. [Crossref] [PubMed]
- Corso CR, Stipp MC, Radulski DR, et al. Fruticuline A, a chemically-defined diterpene, exerts antineoplastic effects in vitro and in vivo by multiple mechanisms. Sci Rep 2020;10:16477. [Crossref] [PubMed]
- Adami ER, Corso CR, Turin-Oliveira NM, et al. Antineoplastic effect of pectic polysaccharides from green sweet pepper (Capsicum annuum) on mammary tumor cells in vivo and in vitro. Carbohydr Polym 2018;201:280-92. [Crossref] [PubMed]
- Adami ER, Corso CR, Turin-Oliveira NM, et al. Polysaccharides from green sweet pepper increase the antineoplastic effect of methotrexate on mammary tumor cells. Int J Biol Macromol 2020;158:1071-81. [Crossref] [PubMed]
- Abdin AA, Soliman NA, Saied EM. Effect of propranolol on IL-10, visfatin, Hsp70, iNOS, TLR2, and survivin in amelioration of tumor progression and survival in Solid Ehrlich Carcinoma-bearing mice. Pharmacol Rep 2014;66:1114-21. [Crossref] [PubMed]
- Bassiony H, Sabet S, Salah El-Din TA, et al. Magnetite nanoparticles inhibit tumor growth and upregulate the expression of p53/p16 in Ehrlich solid carcinoma bearing mice. PLoS One 2014;9:e111960. [Crossref] [PubMed]
- Silva AE, Santos FGA, Cassali GD. Marcadores de proliferação celular na avaliação do crescimento do tumor sólido e ascítico de Ehrlich. Arq Bras Med Veterinária e Zootec 2006;58:658-61.
- Castelló CM. Ultrassonografia do tumor sólido de Ehrlich inoculado em camundongos. Universidade Federal de Goiais; 2017. Available online: https://repositorio.bc.ufg.br/tede/bitstream/tede/7130/5/Dissertação - Carla Martí Castelló - 2017.pdf
- Boone C, Sasaki M, McKee W. Characterization of an in vitro strain of Ehrlich-Lettre ascites carcinoma subjected to many periodic mouse passages. J Natl Cancer Inst 1965;34:725-40.
- Schachtschabel DO, Foley GE. Serial cultivation of Ehrlich ascites tumor cells in hypertonic media. Exp Cell Res 1972;70:317-24. [Crossref] [PubMed]
- Kapoor R, Gundpatil DB, Somani BL, et al. Anticancer Effect of dl-Glyceraldehyde and 2-Deoxyglucose in Ehrlich Ascites Carcinoma Bearing Mice and Their Effect on Liver, Kidney and Haematological Parameters. Indian J Clin Biochem 2014;29:213-20. [Crossref] [PubMed]
- Mishra S, Tamta AK, Sarikhani M, et al. Subcutaneous Ehrlich Ascites Carcinoma mice model for studying cancer-induced cardiomyopathy. Sci Rep 2018;8:5599. [Crossref] [PubMed]
- Lomax CA, Henderson JF. New method for the isolation of drug-resistant clones of Ehrlich ascites tumor cells. J Natl Cancer Inst 1974;52:1291-5. [Crossref] [PubMed]
- Rabinovitz M, Fisher JM. S-Carbamylcysteine inhibition of protein synthesis by Ehrlich ascites tumor cells. J Natl Cancer Inst 1962;28:1165-71.
- Feitosa IB, Mori B, Teles CBG, et al. What are the immune responses during the growth of Ehrlich’s tumor in ascitic and solid form? Life Sci 2021;264:118578. [Crossref] [PubMed]
- Segura JA, Barbero LG, Márquez J. Ehrlich ascites tumour unbalances splenic cell populations and reduces responsiveness of T cells to Staphylococcus aureus enterotoxin B stimulation. Immunol Lett 2000;74:111-5. [Crossref] [PubMed]
- Inzhevatkin EV, Savchenko AA. The metabolic changes in tumor-associated macrophages during cancer grow in mice with Ehrlich ascites carcinoma. Dokl Biochem Biophys 2017;475:277-9. [Crossref] [PubMed]
- Bergami-Santos PC, Mariano M, Barbuto JA. Dual role of polymorphonuclear neutrophils on the growth of Ehrlich ascites tumor (EAT) in mice. Life Sci 2004;75:245-55. [Crossref] [PubMed]
- Mandal D, Lahiry L, Bhattacharyya A, et al. Tumor-induced thymic involution via inhibition of IL-7R alpha and its JAK-STAT signaling pathway: protection by black tea. Int Immunopharmacol 2006;6:433-44. [Crossref] [PubMed]
- Chatterjee S, Das S, Chakraborty P, et al. Myeloid derived suppressor cells (MDSCs) can induce the generation of Th17 response from naïve CD4+ T cells. Immunobiology 2013;218:718-24. [Crossref] [PubMed]
- Molotkov A, Satoh M, Tohyama C. Tumor growth and food intake in interleukin-6 gene knock-out mice. Cancer Lett 1998;132:187-92. [Crossref] [PubMed]
- Fernandes PD, Guerra FS, Sales NM, et al. Characterization of the inflammatory response during Ehrlich ascitic tumor development. J Pharmacol Toxicol Methods 2015;71:83-9. [Crossref] [PubMed]
- Fecchio D, Sirois P, Russo M, et al. Studies on inflammatory response induced by Ehrlich tumor in mice peritoneal cavity. Inflammation 1990;14:125-32. [Crossref] [PubMed]
- Ruiz de Morales J, Vélez D, Subiza JL. Ehrlich tumor stimulates extramedullar hematopoiesis in mice without secreting identifiable colony-stimulating factors and without engagement of host T cells. Exp Hematol 1999;27:1757-67. [Crossref] [PubMed]
- Ibrahim HM, Abdel Ghaffar FR, El-Elaimy IA, et al. Antitumor and immune-modulatory efficacy of dual-treatment based on levamisole and/or taurine in Ehrlich ascites carcinoma-bearing mice. Biomed Pharmacother 2018;106:43-9. [Crossref] [PubMed]
- da Silva IL, Veloso ES, Gonçalves INN, et al. Qa-2 expression levels is related with tumor-infiltrating lymphocytes profile during solid Ehrlich tumor development. Biomed Pharmacother 2017;92:750-6. [Crossref] [PubMed]
- Calixto-Campos C, Zarpelon AC, Corrêa M, et al. The Ehrlich tumor induces pain-like behavior in mice: a novel model of cancer pain for pathophysiological studies and pharmacological screening. Biomed Res Int 2013;2013:624815. [Crossref] [PubMed]
- Violato NM, Rafacho A, Boschero AC, et al. Higher insulin sensitivity and impaired insulin secretion in cachetic solid ehrlich tumour-bearing mice. Horm Metab Res 2014;46:615-20. [Crossref] [PubMed]
- De Oliveira KM. Avaliação dos Efeitos Antitumorais e Hepáticos de Polissacarídeos extraídos do Tucum-do-Cerrado (Bactris setosa Mart) em Camundongos. Federal of Parana University; 2022.
- Kulagina TP, Aripovsky AV, Gapeyev AB. Changes in fatty acid composition of thymus cells, liver, blood plasma, and muscle tissue in mice with solid Ehrlich carcinoma. Biochemistry (Mosc) 2012;77:187-93. [Crossref] [PubMed]
- Hristov P, Tyurin V, Petrov L. Factors of Ehrlich Ascites Tumor resistance to oxidative stress. Acta Physiol Pharmacol Bulg 1995;21:49-55.
- Jiang ZY, Woollard AC, Wolff SP. Lipid hydroperoxide measurement by oxidation of Fe2+ in the presence of xylenol orange. Comparison with the TBA assay and an iodometric method. Lipids 1991;26:853-6. [Crossref] [PubMed]
- Szabados G, Tretter L, Horvath I. Lipid peroxidation in liver and Ehrlich ascites cell mitochondria. Free Radic Res Commun 1989;7:161-70. [Crossref] [PubMed]
- Filipek A. S100A6 and CacyBP/SIP - two proteins discovered in ehrlich ascites tumor cells that are potentially involved in the degradation of beta-catenin. Chemotherapy 2006;52:32-4. [Crossref] [PubMed]
- Donenko FV, Ziganshin RH, Anisimova NY, et al. Identification of serpin (alpha-1-antitrypsin) as serum growth inhibitory factor in murine ehrlich carcinoma by proteomics. Cancer Genomics Proteomics 2010;7:147-56.
- Donenko FV, Kabieva AO, Efferth T. Tumor-specific blood serum factors as determinants of tumor growth. Klin Lab Diagn 2013;(10):50-2, 13-5.
- Gabai VL, Mosina VA, Budagova KR, et al. Spontaneous overexpression of heat-shock proteins in Ehrlich ascites carcinoma cells during in vivo growth. Biochem Mol Biol Int 1995;35:95-102.
- Nielsen D, Maare C, Eriksen J, et al. Expression of P-glycoprotein and multidrug resistance associated protein in Ehrlich ascites tumor cells after fractionated irradiation. Int J Radiat Oncol Biol Phys 2001;51:1050-7. [Crossref] [PubMed]
- Maragno-Correa JM, Patti CL, Zanin KA, et al. Sleep deprivation increases mortality in female mice bearing Ehrlich ascitic tumor. Neuroimmunomodulation 2013;20:134-40. [Crossref] [PubMed]
- Hamasato EK, de Lima AP, de Oliveira AP, et al. Cohabitation with a sick partner increases allergic lung inflammatory response in mice. Brain Behav Immun 2014;42:109-17. [Crossref] [PubMed]
- Machado TR, Alves GJ, Quinteiro-Filho WM, et al. Cohabitation with an Ehrlich tumor-bearing cagemate induces immune but not behavioral changes in male mice. Physiol Behav 2017;169:82-9. [Crossref] [PubMed]
- Morgulis MS, Stankevicius D, Sá-Rocha LC, et al. Cohabitation with a sick cage mate: consequences on behavior and on ehrlich tumor growth. Neuroimmunomodulation 2004;11:49-57. [Crossref] [PubMed]
- Albalawi AE, Althobaiti NA, Alhasani RH, et al. Anti-tumor effects and cellular mechanisms of Pistacia atlantica methanolic extract against Ehrlich solid tumor in mice. Asian Pac J Trop Biomed 2022;12:69-77.
- Sannappa Gowda NG, Shiragannavar VD, Prabhuswamimath SC, et al. Ehrlich Ascites carcinoma mice model for studying liver inflammation and fibrosis. Adv Cancer Biol - Metastasis 2022;4:2667-3940.
- Aysan E, Bayrak OF, Aydemir E, et al. Cancer preventive effects of whole cell type immunization against mice Ehrlich tumors. Asian Pac J Cancer Prev 2013;14:3515-9. [Crossref] [PubMed]
- Alkreathy HM, Alkhatib MH, Al Musaddi SA, et al. Enhanced antitumour activity of doxorubicin and simvastatin combination loaded nanoemulsion treatment against a Swiss albino mouse model of Ehrlich ascites carcinoma. Clin Exp Pharmacol Physiol 2019;46:496-505. [Crossref] [PubMed]
- Badr El-Din NK, Shabana SM, Abdulmajeed BA, et al. A novel kefir product (PFT) inhibits Ehrlich ascites carcinoma in mice via induction of apoptosis and immunomodulation. BMC Complement Med Ther 2020;20:127. [Crossref] [PubMed]
- Morsi DS, El-Nabi SH, Elmaghraby MA, et al. Anti-proliferative and immunomodulatory potencies of cinnamon oil on Ehrlich ascites carcinoma bearing mice. Sci Rep 2022;12:11839. [Crossref] [PubMed]
- Elhady SS, Habib ES, Abdelhameed RFA, et al. Anticancer Effects of New Ceramides Isolated from the Red Sea Red Algae Hypnea musciformis in a Model of Ehrlich Ascites Carcinoma: LC-HRMS Analysis Profile and Molecular Modeling. Mar Drugs 2022;20:63. [Crossref] [PubMed]
- Morgan HJN, Delgado AQ, Saldanha LL, et al. Vochysia tucanorum Mart. butanol fraction presents antitumoral activity in vivo and prevents the installation of cachexia in solid Ehrlich tumor model. BMC Complement Med Ther 2021;21:20. [Crossref] [PubMed]
- Lima LTF, Ganzella FAO, Cardoso GC, et al. l-carvone decreases breast cancer cells adhesion, migration, and invasion by suppressing FAK activation. Chem Biol Interact 2023;378:110480. [Crossref] [PubMed]
- Tamiello CS, Adami ER, de Oliveira NMT, et al. Structural features of polysaccharides from edible jambo (Syzygium jambos) fruits and antitumor activity of extracted pectins. Int J Biol Macromol 2018;118:1414-21. [Crossref] [PubMed]
- Chequin A, Costa LE, de Campos FF, et al. Antitumoral activity of liraglutide, a new DNMT inhibitor in breast cancer cells in vitro and in vivo. Chem Biol Interact 2021;349:109641. [Crossref] [PubMed]
- Galindo CM. O Metabolismo tumoral de chalconas como alvo terapêutico no Câncer de mama. University Federal of Paraná; 2021.
- Bertozzi MM, Saraiva-Santos T, Zaninelli TH, et al. Ehrlich Tumor Induces TRPV1-Dependent Evoked and Non-Evoked Pain-like Behavior in Mice. Brain Sci 2022;12:1247. [Crossref] [PubMed]
- G MS. Cancer Chemoprevention: A Strategic Approach Using Phytochemicals. Front Pharmacol 2021;12:809308. [Crossref] [PubMed]
- Mostafa A, Mohamed Abdelsalam S. Mesenchymal Stem Cells Treatment Aggravates Tumor Growth Regardless Its Route of Administration: An In vivo Study. Asian Pac J Cancer Prev 2022;23:3309-15. [Crossref] [PubMed]
- Nishikawa H, Goto M, Fukunishi S, et al. Cancer Cachexia: Its Mechanism and Clinical Significance. Int J Mol Sci 2021;22:8491. [Crossref] [PubMed]
- Perez IE, Taveras Alam S, Hernandez GA, et al. Cancer Therapy-Related Cardiac Dysfunction: An Overview for the Clinician. Clin Med Insights Cardiol 2019;13:1179546819866445. [Crossref] [PubMed]
- Rajan A, Sullivan R, Bakker S, et al. Critical appraisal of translational research models for suitability in performance assessment of cancer centers. Oncologist 2012;17:e48-57. [Crossref] [PubMed]
- Ribba B, Holford NH, Magni P, et al. A review of mixed-effects models of tumor growth and effects of anticancer drug treatment used in population analysis. CPT Pharmacometrics Syst Pharmacol 2014;3:e113. [Crossref] [PubMed]
- Yates JWT, Fairman DA. How translational modeling in oncology needs to get the mechanism just right. Clin Transl Sci 2022;15:588-600. [Crossref] [PubMed]
- Zeng L, Li W, Chen CS. Breast cancer animal models and applications. Zool Res 2020;41:477-94. [Crossref] [PubMed]
- Cancer models for reverse and forward translation. Nat Cancer 2022;3:135. [Crossref] [PubMed]
- Li Z, Zheng W, Wang H, et al. Application of Animal Models in Cancer Research: Recent Progress and Future Prospects. Cancer Manag Res 2021;13:2455-75. [Crossref] [PubMed]
- Gómez-Cuadrado L, Tracey N, Ma R, et al. Mouse models of metastasis: progress and prospects. Dis Model Mech 2017;10:1061-74. [Crossref] [PubMed]
- Pessina A, Brambilla P, Mocarelli P. Surface antigen on Ehrlich ascites tumor cells. Biomedicine 1980;33:105-9.
Cite this article as: Radulski DR, Stipp MC, Galindo CM, Acco A. Features and applications of Ehrlich tumor model in cancer studies: a literature review. Transl Breast Cancer Res 2023;4:22.