Precision medicine for breast cancer: advances and challenges
In contemporary oncology, powerful tools such as surgery, chemotherapy, and radiation therapy are employed to prolong survival time and improve the quality of life for breast cancer patients. However, due to tumor heterogeneity, the clinical and molecular characteristics of specific breast cancer subtypes remain elusive, necessitating the emergence of precision medicine.
Precision medicine represents a novel medical paradigm for prevention, diagnosis, and treatment, relying on multimodal data, including molecular characteristics and radiomic features. It highlights personalized medicine and also takes genetic, environmental and lifestyle factors into account. Emerging technologies such as high-throughput sequencing, liquid biopsy, spatial omics, three-dimensional (3D) organoids, network analysis and machine learning have enhanced our understanding of the molecular heterogeneity of breast cancer and led to therapy advancement. Precision medicine not only leads to better therapeutic effects at a personal level due to its personalization, differentiation and variability, but also enables the maximum health benefit with minimum resource investment at a population level. By summarizing the advances and future challenges of precision medicine in breast cancer treatment, we hope to highlight its significant impact on clinicians and researchers, and to encourage great adaptation to the era of precision medicine.
Current directions and advances in the era of precision medicine
Accurate molecular classification (Figure 1)
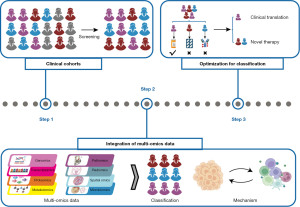
Perou et al. classified breast cancer into several subtypes based on biological characteristics (1). The classification contributed to nearly 40% decrease of total mortality and less treatment-related complications over the past few years, especially for the anti-human epidermal growth factor receptor 2 (HER2) positive breast cancer (2). However, emerging accurate molecular classification has improved treatment specificity and provided benefits to a larger patient population. Contemporary research focuses on refining these classifications to better understand tumor heterogeneity and optimize precision therapy.
Constructing clinical cohorts is essential for accurate molecular classification. Notable cohorts such as The Cancer Genome Atlas (TCGA) cohort, Molecular Taxonomy of Breast Cancer International Consortium (METABRIC) cohort and Clinical Proteomic Tumor Analysis Consortium (CPTAC) cohort are mainly based on western populations. Recently, a large Chinese breast cancer cohort has been established based on the Chinese Breast Cancer Genome Atlas (CBCGA) project (3). These systematic cohorts based on different populations provide new insights into biological heterogeneity and racial specificity, offering valuable resources for targeted therapy.
Multi-omics platforms based on large cohorts have constituted an important direction in precision medicine research. Advancements in technologies like Xenium and artificial intelligence (AI) liberate researches from limited aspects of the central dogma and enable comprehensive analyses of spatial locations and tumor microenvironments, facilitating the integration and distinction of biological behaviors and molecular characteristics.
The application of integrated multi-omics serves two primary purposes. One is to identify statistically significant common features across a large population, expanding the number of patients benefiting from specific treatments. Through systematic multi-omics analysis of the CBCGA cohort, researchers identified more AKT1 mutations, more frequent ERBB2 amplification and higher HER2 protein abundance in the Chinese hormone receptor-positive (HR+)/HER2+ cohort compared to white individuals, revealing anti-HER2 therapy for these patients (3). For HR+/HER2− patients, a subtyping system which classified the tumors into canonical luminal, immunogenic, proliferative and receptor tyrosine kinase (RTK)-driven subtypes provided guidance for individualized treatment (4).
Another is to decipher mechanisms of sensitivity or resistance to specific agents according to the curative effect based on multi-omics integration. A phase Ib/IIa trial (NCT02871791) evaluated the safety, tolerability and clinical benefit of exemestane plus everolimus and palbociclib for cyclin-dependent kinase 4/6 (CDK4/6) inhibitor-resistant metastatic breast cancer (5), exploring resistance mechanisms through whole exome sequencing (WES) and RNA sequencing (RNA-seq) data. Similarly, our team investigated treatment resistance in the luminal androgen receptor (LAR) subtype of triple-negative breast cancer (TNBC). We demonstrated the resistance mechanism by integrating the multi-omics data and deciphering ferroptosis atlas, and uncovered a novel combination strategy for LAR TNBCs (6).
Divergent from the holistic view of the integration of multi-omics, there are still substantial work focusing on a specific omics. This kind of study aims to identify potential anti-tumor targets within tumor cells and explore mechanisms in depth. We previously profiled a metabolomic landscape of TNBC and found the potential oncogenic metabolites such as sphingosine-1-phosphate (S1P) and N-acetyl-aspartyl-glutamate (NAAG) (7). Additionally, radiomics data facilitated non-invasive assessment of intra-tumor heterogeneity in clinical practice (8). By leveraging digital pathology and artificial intelligence (AI), we developed single-cell morphological and topological profiling (sc-MTOP), identifying four breast cancer ecotypes with distinct molecular characteristics and prognoses (9). More recently, we set our sights on the interactions between somatic and germline alterations, expanding the indications of DNA repair-targeted therapy and immunotherapy (10). The work mentioned above demonstrated biological traits from different dimension of omics and further pinpointed therapeutic strategies for different types of breast cancer.
Considering the advancements in breast cancer subtyping systems, continuous optimization for each kind of classification is in desperate need. According to the Fudan University Shanghai Cancer Center (FUSCC) TNBC cohort and CBCGA cohort, distinct subtypes have been identified for TNBCs and HR+/HER2− breast cancers, respectively (4,11). In both studies, we proposed different precision treatment strategies for each subtype, and corresponding clinical trials are currently underway to verify their efficacy and safety. Take FUTURE series trials for example. The phase Ib/II FUTURE trial evaluated the efficacy of subtyping-based therapy for refractory metastatic TNBCs (12). Treatment based on FUSCC classification increased objective response rate from 5–10% to 29.8% compared to conventional chemotherapy. To further expand the use of immunomodulatory subtypes and screen innovative combinational immunotherapy strategies, the phase II FUTURE-C-Plus trial assessed the feasibility of combining famitinib, camrelizumab and chemotherapy in advanced immunomodulatory TNBCs (13). More recently, the phase II FUTURE-SUPER trial confirmed the efficacy and safety of subtyping-based therapy in the first-line treatment of TNBC, further pinpointing a therapeutic strategy for patients with common mutations (14). The median overall survival was 11.3 and 5.8 months, respectively, for the precision treatment and standard chemotherapy arms. These trials reflect the ongoing optimization of subtyping-based therapy. By promoting clinical translation of treatment arms achieving prespecified efficacy boundaries and developing novel therapy for arms with poor outcome, accurate molecular classification and its continual refinement can pave the way for precision treatment.
New drug development and clinical trials: focus on certain therapeutic targets
Apart from accurate molecular classification, research targets potential genomic and transcriptomic targets to combat tumor cells. Representative therapies include small molecule therapeutics targeting specific genetic events, evolving antibody-drug conjugates (ADCs), and immunotherapy that modulates the immune microenvironment and checkpoints.
Genetic mutations can endow tumor cells with unique biological characteristics, rendering them sensitive to small-molecule inhibitors. Patients with BRCA-mutations exhibited impaired DNA damage repair and therefore are sensitive to poly (ADP-ribose) polymerase (PARP) inhibitors. Tumors with PIK3CA mutations can maintain growth despite hormonal suppression, demonstrating resistance to endocrine therapy but benefiting from PI3K inhibitors. Numerous clinical trials are investigating targeted drugs. The phase III OlympiA trial reported an invasive disease-free survival rate of 85.9% in the sub-cohort of olaparib treatment with gBRCA mutation (15). For advanced patients with PIK3CA mutation, the INAVO120 trial, an adaptively randomized phase III study, reported a higher estimated objective response rate for inavolisb plus palbociclib plus fulvestrant treatment (58% vs. 25%) (16).
ADCs exhibited high lethality and a wider therapeutic window, overcoming the limitations of traditional broad-spectrum chemotherapy. The phase III TROPiCS-02 trial shed light on patients with HR+/HER2− advanced or metastatic breast cancer, which pointed out the survival benefit of sacituzumab govitecan over single-agent chemotherapy (17). And the phase III DESTINY-Breast09 trial is challenging the standard first-line treatment options for HER2+ metastatic breast cancer.
Immunotherapy activates the body’s natural defenses to recognize and attack tumor cells. Due to high-immune-infiltration and lack of targetable receptors, immunotherapy is more successful in TNBC. The KEYNOTE 522 trial pointed to increased pathological complete response rate (from 51.3% to 64.8%) and increased event free survival rate (from 72.2% to 81.2%) with the addition of pembrolizumab to a carboplatin-containing chemotherapy backbone in high-risk early-stage TNBCs (18). Additionally, some research focused on cell reinfusion therapy. Our work revealed regional delivery of C-C motif chemokine ligand 19 (CCL19) improved the potency of TNBC immunotherapy in combination with programmed cell death protein 1 (PD-1) blockade through exploration of heterogeneity in dendritic cells (19). Moreover, anti-angiogenic drugs and targeting of tumor innervation are uncovering promising avenues in precision medicine and warrant further investigation.
The challenge for precision treatment of breast cancer
Research challenges
Tumor heterogeneity and evolution
Intra-tumoral heterogeneity provides the fuel for tumor evolution and drug resistance, thus posing significant obstacles to the widespread implementation of precision medicine. In the seesaw between humanity and cancer, more attention should be paid on targeting undiscovered signal transduction pathways since predicting tumor evolution correctly can equip us with more reserved “weapons”.
Precision treatment beyond first-line treatment
Hunting for most suitable treatment at certain stage of cancer is imperative. However, owing to the tumor evolution and heterogeneity, acquired resistance is inevitable to any drug in the foreseeable future. However, research on treatment options for residual disease remains limited.
Metastatic breast cancer management
Metastatic breast cancer is still considered incurable and accounts for over 90% of breast cancer-related death (20). Immunotherapy and ADCs seem to offer new hope. The phase III KEYNOTE-355 trial conferred greater benefit of chemoimmunotherapy for patients whose programmed cell death ligand 1 (PD-L1) combined positive score (CPS) ≥10 (21). Clinical research on ADCs like DESTINY-Breast06 and phase III ASCENT trials confirmed the efficacy of trastuzumab deruxtecan (T-Dxd) and sacituzumab govitecan treatment for metastatic breast cancer separately, moving ADCs towards the first-line treatment (22,23). Identifying biomarkers to determine the beneficiary population for these two types of treatments remains a significant challenge.
Undruggable targets
Achilles’ heel of cancer cells has gradually emerged in public eye thanks to accurate molecular classification and integrated multi-omics analysis. However, the designed targeted arrow is very limited. Some drug targets remain undruggable owing to systemic toxicity, off-target effects or inability to reach an effective concentration, hindering their clinical translation.
Incongruent data from various studies
Inconsistent data across different studies limit the applicability of precision medicine. The variation in efficacy evaluation, classification criteria, and omics data types among institutions and countries poses challenges to data integration, restricting the number of patients benefiting from precision treatment.
Clinical implication
While multi-omics analysis provides comprehensive guidance to target therapy, high testing costs and patients’ unawareness of its necessity hinder its implementation. More effort should be put on discovering more economical, accessible and appliable targets in clinical testing. Radiomics and digital pathology can provide clues for diagnosis or treatment just relying on radiology films or hematoxylin-eosin stained slides. The convenience and low costing of these methods are not only beneficial to the optimization and validation of relevant features in large sample cohorts, but also promote their implementation in real-world clinical practice.
Accessibility of treatments is another key issue. Many new drugs are still in early development stages, which makes patients not accessible to the latest treatments. Additionally, no unified molecular classification system has been developed at present, which leads to inconsistent results and confusion for patients and healthcare providers. Considering this, efforts should be taken to unify or simplify current classification to refine the suitability principle.
Conclusions and future directions
The essence of precision medicine lies in choosing the right treatment at the right time (24). Accurate molecular classification and emerging immunotherapy have ushered in the era of precision oncology. However, challenges like tumor heterogeneity and limited accessibility impede the widespread implementation of precision medicine, and beyond is where we begin.
Maximizing the utility of high-quality cohorts
The establishment of standardized, large sample, multi-center, spatiotemporally paired cohorts provides researchers with valuable insights into the efficacy of specific targeted therapies and expands participant access to the latest treatments. Apart from cohort construction, the trial design is getting more and more attention in precision treatment. Basket and umbrella trials, master platform trials and N-of-1 patient-centric studies are supplanting traditional phase I, II, and III protocols due to their edges on drug approval and molecular-based individualized treatment (25). Considering the requirement of beneficiary population, integrating multi-omics data across large clinical cohorts is a good strategy. In addition, emerging technologies such as organoid and genome editing are useful tools to elucidate mechanisms relative to drug resistance or immune escape.
Interaction, verification and complement within and across different omics
Previous research focused on the discovery of specific features in a certain type of omics, such as gene mutations or overexpression of certain protein. However, the interactive relationship between different traits within certain omics was neglected. Constructing networks comprising co-alterations and mutually exclusive events, as demonstrated in our recent study, sets a model for studying genomic interactions (10).
Different omics datasets can often complement each other, as they capture different insights of the same molecular system. Features derived from radiomics and digital pathology can be verified by genomics and transcriptomics data (8). Proteomics and microbiomics data can complement the genomic and transcriptomic findings in understanding interactions between tumor cells and microenvironment, as well as interactions between tumors and microorganisms (26).
Establishing a precision medicine framework with AI technology
Integrating AI technology into precision medicine has the potential to revolutionize breast cancer therapy. The application of AI in digital pathology can alleviate the burden on pathologists by interpreting markers and uncovering the prognostic value and biological traits of heterogeneity in radiomics (8). When combined with multi-omics data, AI is able to explore tumor therapeutic targets and support clinical decision-making. Additionally, AI demonstrates significant capability in prognosis prediction. The application of support vector machine (SVM) model aids in interpreting the complex relationships between prognostic features.
Two main challenges for AI in precision medicine are reliability and interpretability, commonly referred black-box problem. Deep learning requires a substantial quantity of training data to ensure availability and reliability. Recently, “interpretable” machine learning has emerged as particularly useful for understanding the effects of genetic alterations in precision medicine applications (27).
Integrating new drugs into the existing treatment regimens and optimizing drug combinations
The development of resistance and overlapping intolerable toxic effects can sometimes hinder the application of new drug combinations. The improved therapeutic index of ADCs makes them appealing combination partners. The I-SPY2.2 trial successfully confirmed the efficacy of atopotamab deruxtecan combined with durvalumab, providing a new idea for combined immunotherapy (28). Ongoing clinical trials like DESTINY-Breast07 are exploring the efficacy of T-DXd treatment in early HER2+ breast cancer and optimize combinational regimens with T-DXd and other drugs (29).
Reasonable and effective targeted drug combinations can increase drug sensitivity, delay the development of drug resistance and expand options for breast cancer patients. Undruggable targets deserve to be re-evaluated to further broaden treatment possibilities. For existing drugs, drug repositioning has shown potential in overcoming the bottleneck of drug development and optimizing candidate drugs for new indications. Systematically identification of active combinations as well as the tissues and molecular contexts in which they are most effective advances the field of combination treatments.
Construction of platform and system for precision medicine
Developing and realizing personalized treatment plans requires integrated platforms and operating systems. The Precision Cancer Medical Center, which unites research, education and clinical practice, exemplifies effective clinical translation of treatment breakthroughs. Patient-derived xenograft models and organoids platform for breast cancer have been generated to predict drug responses, facilitating drug discovery and identification of novel treatment options (30). By integrating multi-omics data, AI can explore therapeutic targets and make decisions with clinical decision-support systems (CDSS) (31). Still, these newly established platforms and systems remain in preliminary stages and lack widespread adoption in primary hospitals and local clinics. Future work should optimize diagnosis and treatment systems leveraging AI and deep learning algorithms, aiming to offer integrated care management for a broad breast cancer patient population.
Acknowledgments
Funding: None.
Footnote
Provenance and Peer Review: This article was commissioned by the editorial office, Translational Breast Cancer Research. The article has undergone external peer review.
Peer Review File: Available at https://tbcr.amegroups.org/article/view/10.21037/tbcr-24-35/prf
Conflicts of Interest: All authors have completed the ICMJE uniform disclosure form (available at https://tbcr.amegroups.org/article/view/10.21037/tbcr-24-35/coif). The authors have no conflicts of interest to declare.
Ethical Statement: The authors are accountable for all aspects of the work in ensuring that questions related to the accuracy or integrity of any part of the work are appropriately investigated and resolved.
Open Access Statement: This is an Open Access article distributed in accordance with the Creative Commons Attribution-NonCommercial-NoDerivs 4.0 International License (CC BY-NC-ND 4.0), which permits the non-commercial replication and distribution of the article with the strict proviso that no changes or edits are made and the original work is properly cited (including links to both the formal publication through the relevant DOI and the license). See: https://creativecommons.org/licenses/by-nc-nd/4.0/.
References
- Perou CM, Sørlie T, Eisen MB, et al. Molecular portraits of human breast tumours. Nature 2000;406:747-52. [Crossref] [PubMed]
- Hayes DF. Further Progress for Patients with Breast Cancer. N Engl J Med 2019;380:676-7. [Crossref] [PubMed]
- Jiang YZ, Ma D, Jin X, et al. Integrated multiomic profiling of breast cancer in the Chinese population reveals patient stratification and therapeutic vulnerabilities. Nat Cancer 2024;5:673-90. [Crossref] [PubMed]
- Jin X, Zhou YF, Ma D, et al. Molecular classification of hormone receptor-positive HER2-negative breast cancer. Nat Genet 2023;55:1696-708. [Crossref] [PubMed]
- Gómez Tejeda Zañudo J, Barroso-Sousa R, Jain E, et al. Exemestane plus everolimus and palbociclib in metastatic breast cancer: clinical response and genomic/transcriptomic determinants of resistance in a phase I/II trial. Nat Commun 2024;15:2446. [Crossref] [PubMed]
- Yang F, Xiao Y, Ding JH, et al. Ferroptosis heterogeneity in triple-negative breast cancer reveals an innovative immunotherapy combination strategy. Cell Metab 2023;35:84-100.e8. [Crossref] [PubMed]
- Xiao Y, Ma D, Yang YS, et al. Comprehensive metabolomics expands precision medicine for triple-negative breast cancer. Cell Res 2022;32:477-90. [Crossref] [PubMed]
- Su GH, Xiao Y, You C, et al. Radiogenomic-based multiomic analysis reveals imaging intratumor heterogeneity phenotypes and therapeutic targets. Sci Adv 2023;9:eadf0837. [Crossref] [PubMed]
- Zhao S, Chen DP, Fu T, et al. Single-cell morphological and topological atlas reveals the ecosystem diversity of human breast cancer. Nat Commun 2023;14:6796. [Crossref] [PubMed]
- Lin CJ, Jin X, Ma D, et al. Genetic interactions reveal distinct biological and therapeutic implications in breast cancer. Cancer Cell 2024;42:701-719.e12. [Crossref] [PubMed]
- Jiang YZ, Ma D, Suo C, et al. Genomic and Transcriptomic Landscape of Triple-Negative Breast Cancers: Subtypes and Treatment Strategies. Cancer Cell 2019;35:428-440.e5. [Crossref] [PubMed]
- Jiang YZ, Liu Y, Xiao Y, et al. Molecular subtyping and genomic profiling expand precision medicine in refractory metastatic triple-negative breast cancer: the FUTURE trial. Cell Res 2021;31:178-86. [Crossref] [PubMed]
- Wu SY, Xu Y, Chen L, et al. Combined angiogenesis and PD-1 inhibition for immunomodulatory TNBC: concept exploration and biomarker analysis in the FUTURE-C-Plus trial. Mol Cancer 2022;21:84. [Crossref] [PubMed]
- Fan L, Wang ZH, Ma LX, et al. Optimising first-line subtyping-based therapy in triple-negative breast cancer (FUTURE-SUPER): a multi-cohort, randomised, phase 2 trial. Lancet Oncol 2024;25:184-97. [Crossref] [PubMed]
- Geyer CE Jr, Garber JE, Gelber RD, et al. Overall survival in the OlympiA phase III trial of adjuvant olaparib in patients with germline pathogenic variants in BRCA1/2 and high-risk, early breast cancer. Ann Oncol 2022;33:1250-68. [Crossref] [PubMed]
- Juric D, Kalinsky K, Turner NC, et al. First-line inavolisib/placebo + palbociclib + fulvestrant (Inavo/Pbo+Palbo+Fulv) in patients (pts) with PIK3CA-mutated, hormone receptor-positive, HER2-negative locally advanced/metastatic breast cancer who relapsed during/within 12 months (mo) of adjuvant endocrine therapy completion: INAVO120 Phase III randomized trial additional analyses. J Clin Oncol 2024;42:abstr 1003.
- Rugo HS, Bardia A, Marmé F, et al. Overall survival with sacituzumab govitecan in hormone receptor-positive and human epidermal growth factor receptor 2-negative metastatic breast cancer (TROPiCS-02): a randomised, open-label, multicentre, phase 3 trial. Lancet 2023;402:1423-33. [Crossref] [PubMed]
- Schmid P, Cortes J, Dent R, et al. Overall Survival with Pembrolizumab in Early-Stage Triple-Negative Breast Cancer. N Engl J Med 2024; Epub ahead of print. [Crossref] [PubMed]
- Wu SY, Zhang SW, Ma D, et al. CCL19+ dendritic cells potentiate clinical benefit of anti-PD-(L)1 immunotherapy in triple-negative breast cancer. Med 2023;4:373-393.e8. [Crossref] [PubMed]
- Peng ZH, Jogdeo CM, Li J, et al. Tumor Microenvironment-Responsive Polymeric iRGD and Doxorubicin Conjugates Reduce Spontaneous Lung Metastasis in an Orthotopic Breast Cancer Model. Pharmaceutics 2022;14:1725. [Crossref] [PubMed]
- Cortes J, Rugo HS, Cescon DW, et al. Pembrolizumab plus Chemotherapy in Advanced Triple-Negative Breast Cancer. N Engl J Med 2022;387:217-26. [Crossref] [PubMed]
- Bardia A, Rugo HS, Tolaney SM, et al. Final Results From the Randomized Phase III ASCENT Clinical Trial in Metastatic Triple-Negative Breast Cancer and Association of Outcomes by Human Epidermal Growth Factor Receptor 2 and Trophoblast Cell Surface Antigen 2 Expression. J Clin Oncol 2024;42:1738-44. [Crossref] [PubMed]
- Curigliano G, Hu X, Dent RA, et al. Trastuzumab deruxtecan (T-DXd) vs physician’s choice of chemotherapy (TPC) in patients (pts) with hormone receptor-positive (HR+), human epidermal growth factor receptor 2 (HER2)-low or HER2-ultralow metastatic breast cancer (mBC) with prior endocrine therapy (ET): Primary results from DESTINY-Breast06 (DB-06). J Clin Oncol 2024;42:abstr LBA1000.
- Wahida A, Buschhorn L, Fröhling S, et al. The coming decade in precision oncology: six riddles. Nat Rev Cancer 2023;23:43-54. [Crossref] [PubMed]
- Fountzilas E, Tsimberidou AM, Vo HH, et al. Clinical trial design in the era of precision medicine. Genome Med 2022;14:101. [Crossref] [PubMed]
- Wang H, Rong X, Zhao G, et al. The microbial metabolite trimethylamine N-oxide promotes antitumor immunity in triple-negative breast cancer. Cell Metab 2022;34:581-594.e8. [Crossref] [PubMed]
- Zhao X, Singhal A, Park S, et al. Cancer Mutations Converge on a Collection of Protein Assemblies to Predict Resistance to Replication Stress. Cancer Discov 2024;14:508-23. [Crossref] [PubMed]
- Shatsky RA, Trivedi MS, Omene CO, et al. Rates of pathologic complete response (pCR) after datopotamab deruxtecan (Dato) plus durvalumab (Durva) in the neoadjuvant setting: Results from the I-SPY2.2 trial. J Clin Oncol 2024;42:abstr LBA501.
- Andre F, Hamilton EP, Loi S, et al. DESTINY-Breast07: Dose-expansion interim analysis of T-DXd monotherapy and T-DXd + pertuzumab in patients with previously untreated HER2+ mBC. J Clin Oncol 2024;42:1009. [Crossref]
- Guillen KP, Fujita M, Butterfield AJ, et al. A human breast cancer-derived xenograft and organoid platform for drug discovery and precision oncology. Nat Cancer 2022;3:232-50. [Crossref] [PubMed]
- Somashekhar SP, Sepúlveda MJ, Puglielli S, et al. Watson for Oncology and breast cancer treatment recommendations: agreement with an expert multidisciplinary tumor board. Ann Oncol 2018;29:418-23. [Crossref] [PubMed]
Cite this article as: Liu XY, Yu TJ, Shao ZM. Precision medicine for breast cancer: advances and challenges. Transl Breast Cancer Res 2024;5:35.