Breast cancer shares many epidemiological, lifestyle, and local hormonal and metabolic underpinnings with endometrial and ovarian cancer: a narrative review
Introduction
Background
The three tumor types canonically associated with female reproductive hormones are breast, ovarian, and endometrial cancer (EC). Breast cancer (BC) is far more common and arguably more comprehensively studied as compared to the other hormone-associated tumor types, and there exists an unmet need to understand whether and how lessons from understanding systemic and local influences on BC progression may relate to endometrial and/or ovarian cancer (OC) progression.
Rationale and knowledge gap
Unfortunately, to our knowledge there are no studies that directly compare the impact of these factors head-to-head on breast, endometrial and OC, likely because of the practical impossibilities of comparing the systemic contributors to three tumor types simultaneously. Even so, there is an opportunity to utilize existing information regarding systemic and local contributors to the progression of each tumor type in isolation, for the purpose of generating hypotheses that may potentially generate mechanistic insights in the future. This is particularly timely given evidence, which will be discussed in this review, of common factors related to systemic metabolism, parity, and reproductive hormonal signaling, which may contribute similarly to each tumor type.
Objective
Previously documented intriguing similarities between breast, endometrial and OC beg for a deeper understanding of how the epidemiology and biology of these three female reproductive tumor types may be similar or different. Therefore, this review seeks to examine common threads shared by BC with endometrial and/or OC, and highlight areas of unmet need, with the goal of highlighting targets of opportunity to better understand the basic biology of these tumor types. We present this article in accordance with the Narrative Review reporting checklist (available at https://tbcr.amegroups.com/article/view/10.21037/tbcr-24-39/rc).
Methods
In constructing this narrative review, relevant studies published in English, without any time restriction, were identified by PubMed searches for breast cancer metabolism, ovarian cancer metabolism, and endometrial cancer metabolism (Table 1). No restriction as to study design was considered.
Table 1
Items | Specification |
---|---|
Dates of search | 4/1/2024–7/14/2024 |
Databases searched | PubMed |
Search terms used | Breast cancer metabolism, ovarian cancer metabolism, endometrial cancer metabolism |
Timeframe | Up to July 2024 |
Inclusion criteria | English only |
Selection process | No specific criteria were used for selection of studies |
Any additional considerations | The search was supplemented by a Google search for the same terms; however, no additional references were added |
Epidemiology: demographics and ethnicity
BC makes up about 10% of all cancers, and 18% of cancers in women. It is reported that about 95% of new cases occur in women who are 40 years or older, with the incidence and death rates increasing with age and especially after menopause. Between January 2000 and June 2022, approximately 2.4 million women were reported to have BC from 81 countries (1). In the United States (U.S.), BC is the second most commonly diagnosed cancer and the second leading cause of cancer deaths in women (2). Understanding the influence of ethnicity and demographics on breast and gynecologic cancers is essential for developing effective strategies in prevention, screening/diagnosis, and treatment. Race and ethnicity shape the incidence, presentation, prognosis, and outcomes of these cancers worldwide, as is described subsequently. While the age-standardized incidence rates are higher in White women than Black women, the mortality rate is higher in Black women (3). These differences may stem from various factors, including genetic predispositions, reproductive patterns, hormonal exposures, and socioeconomic conditions, though to our knowledge, controlled studies to assess these possibilities are lacking. In BC specifically, the higher mortality in Black women may relate in part to the fact that triple negative BC (TNBC), the most aggressive BC subtype, particularly in premenopausal women, is more common in Black women (4). Demographic factors such as age, socioeconomic status, and geographic location further influence cancer outcomes, impacting both the aggressiveness of the disease and treatment challenges across different patient groups. Addressing these disparities through targeted research, health equity initiatives, and personalized healthcare approaches is crucial for enhancing outcomes and alleviating the burden of breast and gynecologic cancers in diverse populations.
The differences in BC incidence and prognosis between different racial and ethnic groups are not confined to the U.S. One study reported that compared to North America, there were higher proportions of cases with distant metastatic BC at diagnosis in sub-Saharan Africa. Older age and lower socioeconomic status were some of the factors characterizing the groups with the largest proportion of cases diagnosed at a distant metastatic stage (1). In developing countries, factors like not believing that there is a cure for BC, having a lower educational background and coming from a rural area (often resulting in less access to preventative health care and screening) were associated with diagnosis at a later stage (5). These data suggest that social and/or environmental factors may be strong determinants for late stage of diagnosis in some developing countries.
While less prevalent than BC, both ovarian and ECs also present a substantial public health burden. OC is the sixth most common cancer and the fifth most common cause of cancer death in women from developed countries (6). In 2020, there were about 21,400 new cases of OC in the U.S. OC accounts for about 4% of total deaths due to cancers in American women, while BC accounts for 15% (7). OC occurs mostly in postmenopausal women, with a median age at diagnosis between 60 and 65 years in most developed countries (6). EC is the sixth most common cancer in women (8). EC was diagnosed in more than 415,000 women in 2020 (9) and about 76,000 women die from EC globally each year (10). In the last 30 years, the prevalence of EC has increased by 132%, likely reflecting the increasing prevalence of risk factors like obesity and aging, which will be discussed later in this narrative review, and/or increased screening. Not surprisingly, women from low- and middle-income countries are more likely to die from EC than those from high-income countries (8). Black women have worse outcomes in EC compared to White women and they are less likely to get chemotherapy, radiotherapy or go through hysterectomy (9).
Racial inequities in clinical trials participation
In oncology clinical trials, considering racial and ethnic disparities is equally critical for mitigating inequities in cancer care and promoting health equity. Research consistently demonstrates significant underrepresentation of racial and ethnic minorities in clinical trials, limiting the generalizability of treatment outcomes and exacerbating disparities in accessing innovative therapies. For instance, a recent compilation of 57 trials involving 8,196 participants with TNBC revealed that 74.0% were White, 10.3% Asian, 7.9% African American, 8.0% Hispanic/Latinx, and 4.1% categorized as “combined other”. The inclusion of participants from different racial and ethnic groups in U.S.-based trials comprising 3,348 participants across 36 studies, the distribution included 78.0% White, 12.0% African American, 4.5% Asian, and 5.0% Hispanic/Latinx participants (11). These distributions of clinical trial participants differ markedly from rates of TNBC incidence, which is much less strikingly different between different racial/ethnic groups (Figure 1). Disparities in trial participation are attributed to barriers such as limited awareness about clinical trials, mistrust in medical research, cultural and language barriers, socioeconomic factors, and inadequate access to healthcare resources. Historical injustices and systemic biases within healthcare systems have also contributed to a legacy of mistrust among minority communities, further complicating recruitment efforts.
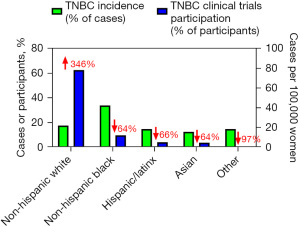
Addressing these challenges requires rigorous efforts to enhance diversity in clinical trial participation through community engagement, culturally sensitive recruitment strategies, educational outreach programs, and partnerships with community organizations. Increasing representation of diverse populations in clinical trials is essential not only for ensuring equitable access to advanced treatments but also for generating comprehensive evidence that reflects the diverse patient population and improves outcomes for all cancer patients.
Reevaluating the impact of obesity on BC risk: the inadequacies of body mass index (BMI) and the need for ethnically inclusive measures of adiposity
Various systemic factors are known to increase the risk of developing BC. Overweight and obesity increased BC risk especially in steroid receptor-expressing BC in postmenopausal women who did not use hormone replacement therapy (13). However, it is important to note that while BMI is usually used as a marker of obesity, BMI is almost certainly not the most accurate measure of obesity-related metabolic dysfunction. An individual with substantial muscle mass and minimal body fat can have the same BMI as an obese person with significantly less muscle (14). This limitation has led to criticism of BMI as an inaccurate measurement tool for predicting cancer risk. Indeed, BMI and visceral fat may correlate differently with tumor gene expression and potentially even outcomes, as our data in lung cancer suggest (15). Additionally, BMI varies among individuals of different ages and activity levels, which can make it misleading. While the World Health Organization (WHO) has provided guidance for interpreting BMI for Asian individuals, who are at higher risk for certain metabolic conditions at lower BMIs, it has not yet offered similar guidance for individuals of Latinx or African descent.
Men and women of Chinese and South Asian origin have a higher amount of visceral adipose tissue at a similar BMI compared to those of European origin. When comparing to Caucasians with the same BMI, Asians have 3% to 5% higher total body fat (16), which may contribute to their high risk of insulin resistance (IR), type 2 diabetes, and their downstream consequences (17). Conversely, some studies have found that African American individuals (especially African American women) have lower body fat and higher lean muscle mass than white individuals at the same BMI, which may result in a lower risk of obesity-related diseases when normalized to BMI. This could be a result of an increase in lean mass, predominantly skeletal muscle, which is associated with improved insulin sensitivity. However, in the U.S., the prevalence of obesity is higher among non-Hispanic African American individuals than non-Hispanic white individuals, leading to a greater overall burden of obesity-related diseases in this group (18).
Impact of obesity on breast and gynecologic cancer risk
Unfortunately, on a population level, BMI is the tool available to the scientific community to understand the association between obesity and cancer. Despite the caveats associated with BMI in predicting individual risk discussed in the previous section, women who are overweight or obese, as defined by BMI, face a higher risk of being diagnosed with BC compared to women who maintain a healthy weight, particularly after menopause (19). Additionally, being overweight can increase the risk of BC recurrence in women who have already been treated for the disease. Women with more visceral fat, as indicated by a high waist-to-hip ratio, are more likely to be diagnosed with hormone-receptor-negative BC, particularly if they are postmenopausal. A possible reason is that subcutaneous fat is involved in estrogen production, which may promote hormone-receptor-positive BC, while visceral fat is more closely related to IR and may be more likely to promote hormone-receptor-negative BC. These findings underscore the critical importance of visceral fat distribution in influencing BC risk and highlight the need for targeted prevention strategies based on individual adiposity patterns.
Like BC, the risk of EC increases with age, BMI, family history and genetic predisposition (9). While the average age at diagnosis is 63 years, EC is now being diagnosed in more young and obese women, with about 57% of EC linked with obesity (15). Women with normal BMI show a 3% lifetime risk of EC; however, for every 5 kg/m2 increase in BMI, there is a 54% increase in risk (8). In a similar vein, obesity increases the risk of OC, with each 5 kg/m2 increase in BMI increasing the lifetime risk of OC, particularly the borderline serous, invasive endometrioid, and invasive mucinous subtypes, by ~10% among women who had never used hormone-replacement therapy (20). While the incidence rate of OC is lower than that of EC and markedly lower than that of BC, OC is most fatal of the three, because it is typically diagnosed at a later stage. A meta-analysis of studies conducted in cohorts from the U.S., Sweden, Germany, Denmark, Australia, China, and Korea concluded that obesity in women with OC is linked with a 17% decrease in survival, with the duration of follow-up up to 32 years (21). While these results are not cancer-specific, and it is likely that the impact of obesity on other aspects of health may have accounted for some of the excess mortality associated with obesity, a separate meta-analysis observed a 10% reduction in progression-free survival in OC patients with obesity. While subject to the same limitations of BMI as a marker of metabolic health as discussed earlier, these results strongly suggest that there may be common factors in the tumor microenvironment (TME) that link obesity and metabolic dysfunction to breast and gynecologic cancers.
Impact of other systemic/hormonal factors on cancer risk
Those women who have a positive family history of BC are 2–4 times more likely to develop cancer, especially those who have the BRCA1 or BRCA2 genes. Women with higher mammographic density have a higher risk of BC. Breast density, a key risk factor for BC, reflects the relative amounts of subcutaneous fat and dense tissues seen on mammograms. Moreover, non-modifiable factors like ethnicity, genetics and race and modifiable ones like exogenous hormones, diet, physical inactivity and female reproductive factors have also been identified as risk factors (22). A relatively unique aspect of BC is that in their primary site, tumor cells are surrounded by adipose tissue, rather than myocytes, epithelial cells, or neurons. In postmenopausal women, adipose tissue is a key source of sex hormones (23). The accumulation of pro-inflammatory cytokines in hypertrophic adipose tissue, as occurs in obesity, and activation of nuclear factor kappa-light-chain-enhancer of activated B cells (NF-κB) in these adipocytes stimulate the activity of the aromatase complex which leads to excessive estrogen synthesis and, in turn, promotes the development of hormone receptor-positive BC (24). Obesity is also strongly associated with IR, hyperinsulinemia, and the production of insulin-like growth factor 1 (IGF-1). BC cells overexpress both the insulin receptor (25) and the IGF-1 receptor (26), and both hormones serve as mitogens in tumor cells. In addition to obesity, IR and hyperinsulinemia are linked with poorer survival in patients with BC (27). Crosstalk between insulin/IGF and estrogen signaling pathways particularly enhances hormone-sensitive BC development (28). Increased insulin and IGF-1 (29) and increased IGF-1 receptor activation are linked with poor survival in patients with BC (30). Early menarche and older age at menopause (i.e., longer duration of exposure to estrogen), as well as having never given birth or older age at first birth, increase BC risk (31). Relatedly, the risk of developing BC has also been shown to be reduced with extended breastfeeding; however, as extended breastfeeding is associated with socioeconomic and geographic factors, and in the absence of a prospective randomized trial to assess the impact of extended breastfeeding on long-term BC risk—which would be essentially impossible to carry out—it is impossible to fully rule out other factors contributing to this association.
Chronic alcohol use has been identified as a risk factor for BC: for every 10 g of pure alcohol consumed every day, there is a 9% increase of BC risk (31). Although there is some evidence that alcohol metabolites are carcinogenic, it is proposed that the link is mostly secondary given that alcohol increases the levels of circulating estrogens by increasing the conversion of androgens to estrogens and reducing estrogen catabolism in the liver (31). Alcohol may also inhibit immune function, thereby facilitating the immune evasion required to establish a tumor. Some studies also point to smoking, during adolescence, to be linked with moderate but statistically significant increase in risk of BC, particularly in women with a family history of BC (32). There is also some evidence that excessive consumption of red and processed meat, saturated fats and sodium increase risk whereas diets high in vegetables, fish and fruits reduce the risk of BC (33), although the role of dietary carcinogens per se, versus effects of dietary composition on IR, body fat quantity and distribution, and inflammation, has not been conclusively established.
There is overlap between reproductive and environmental factors that increase risk or protect from BC, and those that predispose to or protect from gynecologic cancers. Early menarche, late menopause, anovulation [for example, in polycystic ovarian syndrome (PCOS)], and having never given birth also increase EC (8) and OC risk (6). IR and hyperinsulinemia, which are characteristics of obesity, type 2 diabetes, and PCOS, and increase BC risk, also promote EC (8). Hyperinsulinemia is linked with both disordered proliferative endometrium, endometrial hyperplasia, and EC (34) and high IR is associated with a worse prognosis for EC (35). Higher parity, which is inversely correlated with risk of EC, as well as using oral contraceptives, which can reduce gynecologic cancer risk by 30% to 50%, can both help minimize the risk of EC (6,36). However, it should be noted that parity may increase the risk of basal-like but not other subtypes of BC (37). Researchers identified an inverse relationship between drinking coffee and developing EC with a stronger effect in participants with BMI >25 kg/m2 (38), which may point to coffee’s role in being able to reduce concentrations of estrogen and insulin as well as the presence of antioxidants which may have anti-cancer properties. Environmental factors including cigarette smoking, asbestos and talc powder exposures may also increase the risk of OC (39). The fact that these pro-oxidative environmental factors increase the risk of all three tumor types (Figure 2) again points to potential convergent biology between these reproductive cancers.
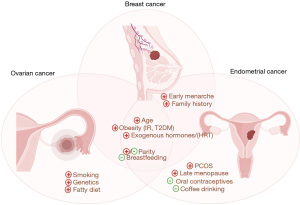
Adipokines in cancer progression: insights from BC and beyond
In cancer treatment, off-target effects can complicate outcomes by impacting non-cancerous tissues and causing adverse side effects. For instance, therapies targeting estrogen receptors (ER) or human epidermal growth factor receptor 2 (HER2) can inadvertently affect other hormonal pathways or cell types expressing these receptors, leading to cardiovascular issues, bone density loss, or immune system suppression. These off-target effects highlight the complexity of cancer treatment and underscore the importance of understanding all factors that influence therapeutic outcomes. Research over the years has revealed the endocrine nature of adipose tissue, which is now recognized as an organ involved in various signaling pathways in (patho)physiology, including inflammation, carcinogenesis, and BC. Adipokines, hormones secreted by adipose tissue, have emerged as significant players in cancer biology. As described in the subsequent section, adipokines can modify the TME (Figure 3), potentially affecting the sensitivity of cancer cells to treatments. This interaction may either enhance or diminish the efficacy of therapies, and may potentially also contribute to off-target effects. Further study of adipokines could help identify mechanisms of adipocyte-mediated chemoresistance, shedding light on how adipose tissue can affect the efficacy of cancer treatments and potentially contribute to treatment resistance.
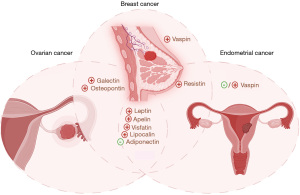
As discussed previously, higher mammographic density is associated with an increased risk of BC. This correlation is likely attributable to the fact that mammographic density is directly associated with the proliferation of stromal and epithelial cells (40), both of which are hallmarks of cancer. This proliferation is linked to the presence of growth-promoting regulatory factors, such as circular RNAs, produced by inflammation-activated fibroblasts, which can trigger carcinogenesis in epithelial cells (41). While circular RNAs, which have recently gained traction in terms of their role in BC, have not yet been associated with mammographic breast density, studies have shown that secretion of other growth factors (collagen, tissue metalloproteinase-3, and IGF-1) is associated with mammographic density (42). Studies also show differences in epithelial composition between dense and non-dense breasts, impacting gene expression related to cell polarity and cell-cell adhesion (43). There is contrasting advice regarding the impact of having higher fat content in the breasts on the risk of BC, with studies showing both a positive (44) and a negative (45) correlation between fat content and BC risk. However, breast reduction mammoplasty is associated with decreased serum leptin levels and improved insulin sensitivity (46). Taken together, these data suggest that adiposity-associated hormones produced in mammary tissue could play a key role in BC development, as will be discussed subsequently.
The adipocyte secretome, comprising proteins, hormones, cytokines, and other factors secreted by adipocytes, plays pivotal roles in metabolic regulation, inflammation, and the modulation of the TME. Studies have identified several adipokines positively associated with BC, including adiponectin, leptin, resistin, visfatin, osteopontin (OPN), apelin, and lipocalin, have been correlated with heightened BC risk, a subset of which have also been implicated in gynecologic cancers. The mechanism by which adipokines possess the ability to directly impact metabolic pathways such as Janus kinase-signal transducer and activator of transcription (JAK-STAT) or phosphoinositide 3-kinase (PI3K), and to modify the TME (47) remains debated. In this section, we will explore the relationships between various adipokines and their roles in endometrial and OC and examine their potential connections with findings from breast and gynecologic cancer research.
Leptin and adiponectin
Leptin and adiponectin are the primary adipocytokines investigated in many hormone-responsive and adiposity-associated cancer studies. Nevertheless, research on the potential molecular mechanisms of these adipokines in driving tumor progression is sparse. Obesity-induced changes in hormone levels, such as increased leptin secretion (in association with increased secretion of insulin and estrogen as discussed previously), with reduced adiponectin expression, play a crucial role in the formation and advancement of breast tumors (48). Leptin has been shown to promote cholesterol biosynthesis; as cholesterol is a critical component for cell membranes, this could be a mechanism by which leptin promotes cancer progression in BC (43) as well as potentially in other tumor types. Indeed, in postmenopausal women, higher leptin levels correlate with increased cancer incidence and altered tumor cell morphology (increased surface roughness) in EC (49,50): postmenopausal women with the highest leptin levels have nearly triple the cancer risk compared to those with the lowest levels (50). Adiponectin is a hormone produced by adipocytes that shows a negative correlation with obesity. Consistent with possible adiponectin-mediated signaling driving worse prognosis in all three tumor types, adiponectin levels correlate negatively (meaning that individuals with low adiponectin levels and/or activity or receptor expression, as are observed in obesity, exhibit accelerated tumor progression) with all three tumor types examined in this review (51). However, the leptin-to-adiponectin ratio (L/A) is suggested to be a more informative indicator of EC risk among postmenopausal women. Compared to leptin or adiponectin levels alone, L/A ratios exhibit a significantly higher odds ratio {6.0 [95% confidence interval (CI): 3.2–11.9] for the L/A ratio, as compared to 3.2 (95% CI: 1.8–5.8) for leptin alone, and 0.5 (95% CI: 0.3–0.9)}, independent of diabetes or age (50). Research in OC has identified significant changes in cytokine secretion profiles in leptin-treated OVCAR-3 and MDAH-2774 cells, highlighting the role of leptin in increasing interleukin (IL)-1 levels through the activation of multiple signaling pathways such as JAK/STAT, p38, mitogen-activated protein kinase (MAPK)/ERK1/2, and PI3K/AKT. Additionally, IL-2 is crucial for regulating mitogenic signals necessary for the differentiation of T and natural killer (NK) lymphocytes, which are key tumor defense effectors (52). The relationship between obesity-associated hormone and OC development is especially evident in postmenopausal women, as shown in a case-control study indicating that Japanese women with low adiponectin in combination with high insulin levels have an increased risk of developing OC (53).
OPN
OPN is highly expressed and secreted by breast tumor cells, promoting the creation of a supportive desmoplastic stroma by stimulating fibroblasts. Its role involves modulating interactions between cells and the extracellular matrix through binding to integrins and CD44 receptors (54). In a similar vein, patients with OC exhibited more than a two-fold increase in median plasma OPN levels as compared to controls (55). While evidence supports OPN’s role in regulating cell proliferation in breast and OC, research on OPN in EC remains limited. However, OPN is increased in patients with endometriosis (56), highlighting the possibility that OPN may promote EC. Further investigation is necessary to elucidate the impact of OPN in this tumor type.
Visfatin
Visfatin is a recently discovered adipokine that has been shown to bind to the insulin receptor and promote glucose utilization in insulin-responsive tissues such as liver and muscle. In OC spheroids, visfatin acts as an anti-apoptotic factor in OC spheroids by regulating mitochondrial activity, thereby enhancing resistance to cell death (57), suggesting visfatin as a potential therapeutic target for ovarian carcinoma. Elevated visfatin levels have also been associated with increased BC risk and poor prognosis, potentially through similar anti-apoptotic mechanisms to what has been observed in OC (58). Factors such as obesity, lipolysis, and IR are also associated with increased serum visfatin levels in EC (50).
Apelin
Apelin, recognized for its role in enhancing insulin sensitivity and regulating glucose metabolism, has emerged as a promising therapeutic target for managing type 2 diabetes. However, elevated apelin expression linked to obesity has been found to exacerbate BC progression, contributing to what is known as the obesity paradox (59). Apelin, predominantly found in the heart, lungs, and mammary glands, plays various physiological roles including apoptosis, inflammation, and tumor proliferation (60). In OC, apelin facilitates metastasis and progression, influencing critical processes like cell proliferation, energy metabolism, and angiogenesis (61). Elevated circulating apelin levels have also been linked to an increased risk of EC development in obese women (62). Thus, apelin represents yet another lipokine that links obesity to an increased risk of each of the three tumor types examined in this review.
Lipocalin
Lipocalin levels increase with diet-induced obesity, contributing to elevated serum levels of both lipocalin and vascular endothelial growth factor (VEGF), which promote angiogenesis in BC (63). The levels of lipocalin-2 were higher in patients with BC compared to the control subjects (64). Lipocalin-2 stimulates BC progression by triggering the epithelial-mesenchymal transition (EMT) in BC cells, and elevated levels may be detected in the urine of metastatic BC patients (65). Within the lipocalin family, lipocalin-2 is notably elevated in patients with leiomyomas, which are more prevalent in pre-menopausal women compared to those with normal endometrium, likely due to higher estrogen levels in leiomyoma patients (66). In chronic kidney disease patients with elevated creatinine levels, a study identified a serum concentration of lipocalin-2 above 160 ng/mL as a potential cut-off point distinguishing benign endometrial changes from EC (66). Lipocalin-2 promotes tumor progression in OC cells through activation of the ERK/GSK3β/β-catenin signaling pathway (67). This pathway has been implicated in numerous tumor types, suggesting a broader impact across different types of cancer, including BC.
Resistin
Elevated resistin levels have been associated not only with increased risk of coronary artery disease in postmenopausal women but also with larger BC tumor sizes in patients with a BMI over 25 kg/m2 (68). In OC, research indicates a significant correlation between elevated resistin levels and poor prognosis, although mechanistic studies specifically investigating resistin’s effects in individuals with obesity remain limited. In patients with EC, there is a significantly higher amount of serum resistin levels when compared to those without the disease (68), although more research will be required to determine any potential causative role for resistin in accelerating the disease.
Galectins
In HER2-positive BC cells, galectin-3 has been implicated in enhancing cancer aggressiveness by activating the PI3K/AKT signaling pathway and promoting stemness via Notch1 signaling activation. These processes collectively contribute to heightened resistance (69). Additionally, galectin-1 levels are elevated in postmenopausal patients and in the serous subtype of epithelial ovarian tumors in OC, underscoring parallels and potential shared mechanisms between breast and OCs (70). Additionally, inhibiting galectin-3 reverses resistance to the monoclonal antibody trastuzumab (Herceptin) in HER2-positive BC cells, indicating potential synergy with trastuzumab therapy for patients (69). Although galectins have been associated with inflammation and endometriosis, there seems to be limited data on how galectins affect EC patients.
Vaspin
Adipokines, including those involved in regulating insulin sensitivity and inflammatory processes, are pivotal in linking insulin levels to BC progression. In overweight BC patients compared to those of normal weight, elevated serum vaspin levels were found in Asian women, suggesting a potential role for increased vaspin in promoting the progression of TNBC by modulating the micro-RNA-33a-5p/a/b-hydrolase domain containing 2 pathway (71). This pathway involves microRNAs related to cellular processes such as proliferation, inflammation, stress response, migration, invasion, differentiation, and apoptosis. Vaspin has also emerged as a promising biomarker in gynecology, especially for evaluating patients with abnormal endometrial bleeding. Research indirectly supports vaspin’s role as a modulator of IR commonly observed in obese patients, which is also pertinent in BC research due to its implications for metabolic dysregulation and cancer progression (72). Although lower levels of circulating adiponectin and vaspin have been found to be associated with an increased risk of developing EC in postmenopausal women, there are data depicting that high vaspin levels may be an independent marker for the presence of EC (73). Further investigation into vaspin levels in epithelial OC warrants additional research and may function as a link to EC. Animal studies indicate that ovarian vaspin expression fluctuates throughout the estrous cycle and is modulated by gonadotropins, insulin, IGF-1, and steroid hormones. Notably, these factors regulate vaspin expression by activating multiple kinases, such as STAT3, MAP3/1, and PI3K/AKT. While data on vaspin levels and OC is lacking, the expression of vaspin mRNA in both white adipose tissue and serum is increased in those with PCOS (74). Additionally, treatment with metformin is linked to reduced serum vaspin levels in women diagnosed with PCOS (73). Further exploration of vaspin’s potential as a biomarker could aid in identifying individuals at increased risk of cancer recurrence, including breast and gynecologic cancer, thereby facilitating tailored clinical interventions.
These discoveries underscore the pivotal role of adipocyte-secreted factors in promoting cancer progression and support the exploration of innovative therapeutic strategies targeting adipokines to improve treatment efficacy and minimize off-target effects in BC patients. By conducting a comparative analysis across gynecologic cancers, it becomes evident that investigating adipokine-mediated pathways is crucial for advancing targeted therapies and refining prognostic approaches in BC research and treatment. These similarities underscore the interconnected nature of adipokine-mediated pathways in cancer biology and illuminate promising avenues for targeted therapies across diverse types of gynecological cancers.
Local factors: tumor metabolism
BC metabolism
BC cells exhibit altered metabolism, characterized by high glucose consumption and high glycolysis leading to excess lactate production, a phenomenon known as the Warburg effect. Glycolysis is particularly important in tumor cells because in addition to generating small amounts of energy, glycolysis produces key intermediates in cell division. Glucose transporters (GLUTs) allow the passage of glucose from outside the cell across the hydrophobic cell membrane and into the cells. There are 14 members of the GLUT family in humans and different GLUTs have specific roles and have different affinities for glucose and other sugars. While glucose is the main substrate for GLUT, they can also transport other substrates. Overexpression of GLUTs (GLUT1–6 & 12) (75) is common in tumor cells. The insulin unresponsive GLUT1 expression is linked with higher grade and poorly differentiated tumors, high proliferation rates and aggressiveness in BC (76,77). Insulin responsive GLUT4 mRNA and protein are expressed in BC tissue (78) and inhibiting GLUT4 reduces basal glucose uptake and induces metabolic reprogramming in BC cells and over time leads to inhibiting cell proliferation (79).
BC cells also exhibit increased expression of glycolysis-related enzymes. Hexokinase 2 (HKII), which phosphorylates glucose in the first rate-limiting step of aerobic glycolysis, is overexpressed in about 79% of breast tumors and is correlated with increased histologic grade and proliferative activity (80). C-Myc, an oncogene that is a master regulator of cancer cell metabolism, activates phosphofructokinase 1 (PFK-1) which is a key enzyme in glycolysis and is correlated with HER2 positive expression and poor prognosis (80). Also, 6-phosphofructo-2-kinase/fructose-2,6-biphosphatase 3 enzyme (PFKFB3) expression is linked with the expression of VEGF-a in BC which promotes angiogenesis and distant metastasis (80). Lactate, which is produced from glycolysis, is transported in and out of cells by monocarboxylate transporter (MCT), and MCT1 overexpression in BC is linked with poor prognosis (27), again emphasizing the key role of glycolysis in BC progression.
Signaling pathways including the PI3K/AKT pathway, AMP-activated protein kinase (AMPK), MAPK, and mammalian target of rapamycin (mTOR) pathways are activated in BC and control the overexpression of glycolysis-related enzymes and GLUTs in BC (80). Also, the activation of transcription factors that are associated with glycolysis like c-Myc, tumor protein 53 (p53) and hypoxia-inducible factor-1 (HIF-1) help to regulate glucose metabolism in cancer cells. Estrogen increases c-Myc which in turn increases the gene expression of GLUT, HK and PFK. This is relevant to most BC, considering that ~80% of BC tumors express the ER. p53 is a tumor suppressor and mutations in p53 is linked with increased glycolysis through the activation of GLUT1, GLUT3 and GLUT4. HIF-1a, which is activated by hypoxia, is overexpressed in BC and promotes a metabolic shift to glycolysis by increasing the expression of glycolysis-related molecules including HKII, PFK-1, lactate dehydrogenase (LDH) A, GLUT1 and GLUT3 as well as suppressing mitochondrial function by activating pyruvate dehydrogenase kinase-1 (80).
The enzyme pyruvate kinase isozyme M2 (PKM2) is expressed in the TME of many cancer types and is associated with poor prognosis and clinical outcomes especially in BC. Interestingly, its expression is positively correlated with sensitivity to chemotherapy drugs in BC cells (81). Conversely, downregulation of PKM2 was observed to reduce the signal transducer and activator of transcription 3 (STAT3) pathway and pSTAT3 expression and this was observed to cause the inhibition of gene transcription and suppression of breast tumor proliferation (82). Given that tumors have high glycolytic rates, glucose often presents a substrate limitation on cell division and thus, cancer cells use other means to avoid glucose starvation. Researchers show that both lactate and glutamine support nicotinamide adenine dinucleotide phosphate (NADPH) production by isocitrate dehydrogenase 1 (IDH1) and malic enzyme (ME1) respectively under glucose-deprived conditions. In 4T1 murine breast tumors, knockdown of ME1 slows down tumor growth in vivo and combined ME1/IDH1 knockout better strongly suppresses tumor growth (83).
BC is comprised of tumor stroma consisting of cancer associated fibroblasts (CAFs), cancer associated adipocytes (CAAs) and immune cells, and these cells play a key role in tumor progression. Researchers isolated fibroblasts from patients with and without BC and showed that CAFs, but not non-cancer-associated fibroblasts, exhibit a metabolic shift to produce lactate and pyruvate which promote biosynthetic pathways in cancer cells. They also identified that chronic hypoxia induces epigenetic reprogramming of HIF1-1a and some glycolytic enzymes to sustain the glycolytic phenotype of these CAFs (84).
Although cancer cells are known to consume more glucose to undergo aerobic glycolysis due to their altered metabolism, research shows that hyperinsulinemia, rather than hyperglycemia in diabetes is linked with increased risk of developing cancer and its progression, as discussed in the “Impact of other systemic/hormonal factors on cancer risk” section, and explored further from a molecular perspective in this section. IGF-1 is a mitogen and inhibits apoptosis (85). High expression of the IGF-1-receptor in tumor cells (86) and in cancer stem cells (87) is correlated with tumor size, lymph node involvement, and prognosis in patients with BC by activating tumor MAPK and PI3K pathway signaling (88). In a feed-forward mechanism, hyperinsulinemia reduces concentrations of IGF binding protein 1 (GFBP-1) and thus increases bioactive concentrations of IGF-1. To study the specific molecular effects of insulin signaling on the development of BC, researchers developed a transgenic [MCK-KR-hIGF-IR (MKR)] mouse model where female mice overexpressing a dominant-negative IGF-1R specifically in skeletal muscle develop hyperinsulinemia. They observed that these MKR mice exhibit accelerated tumor growth as compared to wild-type controls, and lung metastasis was simultaneously increased (89,90). Blocking the PI3K/AKT/mTOR pathway in this model reduces primary tumor growth, confirming the role of mitogenic insulin signaling in driving BC growth. Injecting human cell lines with the insulin receptor silenced by short hairpin RNA into the MKR mice decreased cancer cell growth and metastasis and also suppressed the EMT in cancer cells (90). Together, these findings highlight the crucial role of the insulin receptor signaling pathway in BC progression.
Based on these results implicating insulin as a BC driver through the canonical insulin signaling pathway, Varkaris et al. investigated compounds able to promote PI3Ka activation in PI3KCA-mutant cancers (91). They identified a compound, RLY-2608, which is an allosteric mutant-selective inhibitor of PI3Ka and it inhibited tumor growth in PIK3CA-mutant xenograft models with little effect on insulin. It also led to tumor responses in two patients who were diagnosed with advanced hormone receptor-positive BC with kinase or helical domain PIK3CA mutations with no toxicity. In a conceptually similar vein, researchers combined both polydatin (PD), which has anti-inflammatory and anti-tumor activities, and 2-deoxy-D-glucose (2-DG), an inhibitor of glycolysis, to treat the BC cell lines MCF-7 and 4T1. These drugs synergized to slow cell proliferation and cause apoptosis by inhibiting the ROS/PI3K/AKT/HIF-1a/HK2 signaling axis. There was also a decrease in intracellular ROS levels, and deletion of HK2 (which catalyzes the first step in glycolysis, the only step in which glycolysis can remain intact in cells treated with 2-DG) increased the anti-cancer activity of 2-DG (92).
The BTB and CNC homology1 (BACH1) is a heme-binding transcription factor that is highly expressed in the tumors of TNBC patients, and it targets mitochondrial metabolism. It acts to reduce the amount of glucose used in the TCA cycle, and to negatively regulate the transcription of electron transport chain (ETC) genes. When BACH1 expression is reduced by shRNA or hemin degradation, this sensitizes cells to ETC inhibitors including metformin and expression of BACH1 in heme-resistant BACH1 mutant cells expressing a short hairpin RNA for BACH1 rescues the BACH1 phenotype and restores metformin resistance in hemin-treated cells and tumors (93).
Most BC cells exhibit high lipid synthesis and uptake, perhaps unsurprisingly considering the fact that BC arises in a lipid-rich microenvironment, but not typical of tumors. HER2+ BC cells show high levels of triacylglycerols (TGs), phosphatidylcholine (PC) and phosphatidylethanolamine (PE) (94). In TNBC, MYC or JAK/STAT3-regulated fatty acid (FA) oxidation promotes tumor growth and chemoresistance and may serve as therapeutic targets. Underscoring a critical role for FA metabolism in BC progression, treating HER2+ cells with palmitate (C16:0) leads to AMPK activation and inhibition of FA synthesis which then promotes apoptosis (95). FA synthase (FASN) is an enzyme that catalyzes FA synthesis. HER2+ BC cells have increased FASN through the activation of PI3K while FASN inhibition proves to be more effective on HER2-overexpressing breast epithelial cells (96). In HER2-overexpressing BC cells, using a selective FASN inhibitor leads to apoptosis and reduces HER2+ tumors in in vivo models (97). High plasma erucic acid levels, a monosaturated FA, were linked with reduced risk for HR+ BC while high levels of saturated pentadecanoic FA increase the risk of HR+ BC (98). Metabolomics analysis detected high choline metabolites and glycerophospholipid levels in TNBC tumors from Asian women (99), and high levels of phosphatidylcholines is linked with TNBC recurrence (100). Tissue lipid content may be driven by genetics: BC patients with a BRCA1 mutation have higher triglycerides and unsaturated lipids in their breast tissue, while patients with a BRCA2 mutation have higher levels of cholesterol as well as higher unsaturated lipids (101).
Gynecologic cancer metabolism
Endometrial and OC metabolism share many similarities to BC metabolism, suggesting convergent, hormone-dependent mechanisms that may underlie these three tumor types. Like BC cells, GLUT1 is overexpressed in EC tissues, suggesting its relevance in glucose transport in these tissues which may be related to tumor differentiation. Poorly differentiated endometrial tumors have high GLUT1 expression compared to well-differentiated tissues (102). Both estrogen and progesterone have been identified as upstream regulators of GLUT1, however estrogen is a much stronger regulator. Relatedly, while GLUT3 mRNA expression is lower compared to GLUT1 in EC, GLUT3 is higher in ER/progesterone receptor (PR)-positive EC than in ER/PR negative EC (103). Concordantly, ovarian tumor cells also overexpress GLUT1, which serves as a poor prognostic marker in patients (104), but to our knowledge there is little evidence for GLUT3 as a key mediator of glucose metabolism, or of prognosis, in OC.
High glucose levels also directly enhance the expression of GLUT1 and GLUT3 by modulating AMPK/mTOR/s6 signaling in EC cells (105). There is also evidence that increased glucose can upregulate the level of ER-mediated GLUT4 and facilitate the expression of VEGF/VEGFR, which increases the viability and invasion of exfoliated cancer cells (ECCs) (106). Hypoxia is also known to increase GLUT1 and GLUT3 in endometrial stromal cells (107). Various cytokines have also been identified to synthesize GLUTs. For example, IL-7 can upregulate reactive oxygen species (ROS) which signal through the PI3K/AKT/mTOR pathway to upregulate GLUT1 expression in EC (108). From 68 patient samples of EC tumors and normal tissues collected, researchers found that kinesin family member C1 (KIFC1) was highly upregulated in EC tumors, and this was correlated with poor prognosis in patients. Overexpression of KIFC1 increased cell viability of Ishikawa and HEC-1B cells, reduced the apoptotic rate, increased cell cycle, increased uptake of glucose and lactate production, ATP contents and LDH activity while knockdown inhibited tumor growth in vivo. KIFC1 also increased the expression of several glycolytic genes (GLUT1, HK2, c-Myc and LDH) (109). This suggests that regulatory points at both glucose transport and glucose metabolism are capable of promoting tumor progression in EC cells.
Like BC and EC, some GLUTs are also overexpressed in OC (110). GLUT1 expression correlates with the clinical stage of the disease (111), with a gradual increase in GLUT1 expression seen from borderline to malignant tumors as well as from well-differentiated to poorly-differentiated OC (112). In contrast, neither benign lesions nor normal ovarian epithelial cells show significant GLUT1 protein expression (104) suggesting a requirement for GLUT1 in the development and progression of OC but not in normal ovarian physiology. HIF has been shown to modulate GLUT1 expression in OC and this points to hypoxia’s role in regulating GLUT1 (113,114). TP53 mutations are also known to contribute to the upregulation of GLUT1 in OC (75). While some studies show GLUT3 is expressed in OC and correlates with proliferation potential (111,115), others observe that GLUT3 expression was not significantly correlated with OC (113).
Dysregulated lipid metabolism leads to metabolic changes which enhance the aggressiveness of OC. Proliferating OC tumor cells have a high dependence on high FA metabolism. It is notable that high lipid metabolism is a signature of OC like it is in BC, considering the very different microenvironment (predominantly muscle versus fat) in the normal endometrium as compared to breast. Adipokines such as IL-6, IL-8, MCP-1, TIMP-1 and adiponectin in the OC TME promote cancer cell growth and division by activating FA production in adipocytes (109). IL-17A, a proinflammatory cytokine, promotes growth and metastasis of OC by regulating FA uptake in cancer cells. IL-17A activates STAT3 phosphorylation to enhance expression of FA binding protein-4 expression which enhances FA oxidation and, in turn, increases OC proliferation (116). Adipokines also regulate OC progression by regulating the PI3K/AKT, AMPK and PPAR signaling pathways (117). Polyunsaturated FAs like linoleic acid accumulate in the OC microenvironment and activate PPARb/g signaling in tumor-associated macrophages (TAMs) (118). SIK2, upregulated in adipocyte-rich metastatic deposits, upregulates PI3K- and ACC1-mediated FA oxidation and, in turn, promotes omental metastasis (119).
Given these promising results, different agents targeting FA metabolism have been explored for the treatment of OC. C75 and G28UCM, both synthetic inhibitors of FASN, act by inhibiting lipogenesis and downregulating oncogenic PI3K-AKT signaling pathway (120) to decrease cell growth and induce apoptosis in human OC cells. C93 which is also a FASN inhibitor induced apoptosis and mitigate cisplatin resistance in OC (121). Cerulenin, which inhibits FA biosynthesis in a tumor xenograft model of OC, was found to potentiate the anti-tumor immune responses of cytotoxic T cells and thus inhibit tumor growth in the xenograft models of OC (122). Orlistat, an anti-obesity drug which inhibits FA metabolism in cancers, potentiated the growth-inhibitory effects of cisplatin against platinum-resistant OC cells and induced necrosis and apoptosis in both mouse and human OC cells (123). Cisplatin-resistant cells showed increased FA uptake followed by decreased glucose uptake and lipogenesis, suggesting reprogramming from glucose to FA dependent anabolic and energy metabolism associated with chemotherapy resistance. This increase in FA uptake promotes cancer cell survival and cisplatin-induced oxidative stress by promoting beta-oxidation. Thus, when beta-oxidation is blocked by a small molecule inhibitor combined with cisplatin or carboplatin, these synergistically suppress OC proliferation in vitro and in growth of patient-derived xenografts in vivo (124).
Summary
Taken together, to briefly summarize the current literature on the role of metabolism in female cancers, the role of GLUT1- and to a lesser extent GLUT3-dependent glucose metabolism is largely conserved across breast, endometrial, and OC. FA metabolism, while seemingly also important in all three tumor types, appears to exert its effects in different ways: FA oxidation seems to play an important role in promoting breast and OC progression, while in EC, adipocyte-derived cytokines appear to play a more critical role. However, most of these findings rely primarily on gene expression and pharmacologic interventions in vitro. Therefore, further studies will be required, ideally using gold-standard isotope tracer techniques, to dynamically parse the role and regulation of glucose, lipid, and other substrate metabolism in breast, ovarian, and EC cells, and the potential hormonal and/or environmental dependence and targetability of these processes.
Conclusions
While breast, endometrial, and OCs share some common epidemiological and lifestyle risk factors, such as the influence of obesity and reproductive history, each type has unique local tumor characteristics that drive its development and progression. BC, with its distinct metabolic reprogramming and microenvironment interactions, serves as a key example of how local factors can significantly impact cancer behavior. Understanding these differences is crucial for developing targeted prevention and treatment strategies for each cancer type.
Acknowledgments
None.
Footnote
Reporting Checklist: The authors have completed the Narrative Review reporting checklist. Available at https://tbcr.amegroups.com/article/view/10.21037/tbcr-24-39/rc
Peer Review File: Available at https://tbcr.amegroups.com/article/view/10.21037/tbcr-24-39/prf
Funding: None.
Conflicts of Interest: All authors have completed the ICMJE uniform disclosure form (available at https://tbcr.amegroups.com/article/view/10.21037/tbcr-24-39/coif). The authors have no conflicts of interest to declare.
Ethical Statement: The authors are accountable for all aspects of the work in ensuring that questions related to the accuracy or integrity of any part of the work are appropriately investigated and resolved.
Open Access Statement: This is an Open Access article distributed in accordance with the Creative Commons Attribution-NonCommercial-NoDerivs 4.0 International License (CC BY-NC-ND 4.0), which permits the non-commercial replication and distribution of the article with the strict proviso that no changes or edits are made and the original work is properly cited (including links to both the formal publication through the relevant DOI and the license). See: https://creativecommons.org/licenses/by-nc-nd/4.0/.
References
- Benitez Fuentes JD, Morgan E, de Luna Aguilar A, et al. Global Stage Distribution of Breast Cancer at Diagnosis: A Systematic Review and Meta-Analysis. JAMA Oncol 2024;10:71-8. [Crossref] [PubMed]
- Cancer Facts for Women | Most Common Cancers in Women [Internet]. [cited 2024 Nov 11]. Available online: https://www.cancer.org/cancer/risk-prevention/understanding-cancer-risk/cancer-facts/cancer-facts-for-women.html
- Jatoi I, Sung H, Jemal A. The Emergence of the Racial Disparity in U.S. Breast-Cancer Mortality. N Engl J Med 2022;386:2349-52. [Crossref] [PubMed]
- McCarthy AM, Friebel-Klingner T, Ehsan S, et al. Relationship of established risk factors with breast cancer subtypes. Cancer Med 2021;10:6456-67. [Crossref] [PubMed]
- Jedy-Agba E, McCormack V, Olaomi O, et al. Determinants of stage at diagnosis of breast cancer in Nigerian women: sociodemographic, breast cancer awareness, health care access and clinical factors. Cancer Causes Control 2017;28:685-97. [Crossref] [PubMed]
- Ali AT, Al-Ani O, Al-Ani F. Epidemiology and risk factors for ovarian cancer. Prz Menopauzalny 2023;22:93-104. [Crossref] [PubMed]
- Siegel RL, Miller KD, Fuchs HE, et al. Cancer statistics, 2022. CA Cancer J Clin 2022;72:7-33. [Crossref] [PubMed]
- Crosbie EJ, Kitson SJ, McAlpine JN, et al. Endometrial cancer. Lancet 2022;399:1412-28. [Crossref] [PubMed]
- Makker V, MacKay H, Ray-Coquard I, et al. Endometrial cancer. Nat Rev Dis Primers 2021;7:88. [Crossref] [PubMed]
- Urick ME, Bell DW. Clinical actionability of molecular targets in endometrial cancer. Nat Rev Cancer 2019;19:510-21. [Crossref] [PubMed]
- Rao D, Brabant P, Chau I, et al. International Journal of Cancer Care and Delivery 2023;2024: [Crossref]
- Du XL, Li Z. Incidence trends in triple-negative breast cancer among women in the United States from 2010 to 2019 by race/ethnicity, age and tumor stage. Am J Cancer Res 2023;13:678-91. [PubMed]
- Suzuki R, Orsini N, Saji S, et al. Body weight and incidence of breast cancer defined by estrogen and progesterone receptor status--a meta-analysis. Int J Cancer 2009;124:698-712. [Crossref] [PubMed]
- Silveira EA, Kliemann N, Noll M, et al. Visceral obesity and incident cancer and cardiovascular disease: An integrative review of the epidemiological evidence. Obes Rev 2021;22:e13088. [Crossref] [PubMed]
- Leitner BP, Givechian KB, Ospanova S, et al. Multimodal analysis suggests differential immuno-metabolic crosstalk in lung squamous cell carcinoma and adenocarcinoma. NPJ Precis Oncol 2022;6:8. [Crossref] [PubMed]
- Deurenberg P, Deurenberg-Yap M, Guricci S. Asians are different from Caucasians and from each other in their body mass index/body fat per cent relationship. Obes Rev 2002;3:141-6. [Crossref] [PubMed]
- Gujral UP, Pradeepa R, Weber MB, et al. Type 2 diabetes in South Asians: similarities and differences with white Caucasian and other populations. Ann N Y Acad Sci 2013;1281:51-63. [Crossref] [PubMed]
- Hales CM, Carroll MD, Fryar CD, et al. Prevalence of Obesity and Severe Obesity Among Adults: United States, 2017-2018. NCHS Data Brief 2020;1-8. [PubMed]
- Gravena AAF, Romeiro Lopes TC, Demitto MO, et al. The Obesity and the Risk of Breast Cancer among Pre and Postmenopausal Women. Asian Pac J Cancer Prev 2018;19:2429-36. [PubMed]
- Olsen CM, Nagle CM, Whiteman DC, et al. Obesity and risk of ovarian cancer subtypes: evidence from the Ovarian Cancer Association Consortium. Endocr Relat Cancer 2013;20:251-62. [Crossref] [PubMed]
- Protani MM, Nagle CM, Webb PM. Obesity and ovarian cancer survival: a systematic review and meta-analysis. Cancer Prev Res (Phila) 2012;5:901-10. [Crossref] [PubMed]
- Ahmad A, editor. Breast Cancer Metastasis and Drug Resistance: Challenges and Progress [Internet]. Cham: Springer International Publishing; 2019 [cited 2024 Jul 10]. (Advances in Experimental Medicine and Biology; vol. 1152). Available online: http://link.springer.com/10.1007/978-3-030-20301-6
- Grodin JM, Siiteri PK, MacDonald PC. Source of estrogen production in postmenopausal women. J Clin Endocrinol Metab 1973;36:207-14. [Crossref] [PubMed]
- Smolarz B, Nowak AZ, Romanowicz H. Breast Cancer-Epidemiology, Classification, Pathogenesis and Treatment (Review of Literature). Cancers (Basel) 2022;14:2569. [Crossref] [PubMed]
- Papa V, Pezzino V, Costantino A, et al. Elevated insulin receptor content in human breast cancer. J Clin Invest 1990;86:1503-10. [Crossref] [PubMed]
- Papa V, Gliozzo B, Clark GM, et al. Insulin-like growth factor-I receptors are overexpressed and predict a low risk in human breast cancer. Cancer Res 1993;53:3736-40. [PubMed]
- Srinivasan M, Arzoun H, Gk LB, et al. A Systematic Review: Does Insulin Resistance Affect the Risk and Survival Outcome of Breast Cancer in Women? Cureus 2022;14:e21712. [Crossref] [PubMed]
- Lueprasitsakul P, Latour D, Longcope C. Aromatase activity in human adipose tissue stromal cells: effect of growth factors. Steroids 1990;55:540-4. [Crossref] [PubMed]
- Law JH, Habibi G, Hu K, et al. Phosphorylated insulin-like growth factor-i/insulin receptor is present in all breast cancer subtypes and is related to poor survival. Cancer Res 2008;68:10238-46. [Crossref] [PubMed]
- Atoum MF, Alzoughool F, Al-Hourani H. Linkage Between Obesity Leptin and Breast Cancer. Breast Cancer (Auckl) 2020;14:1178223419898458. [Crossref] [PubMed]
- Nardin S, Mora E, Varughese FM, et al. Breast Cancer Survivorship, Quality of Life, and Late Toxicities. Front Oncol 2020;10:864. [Crossref] [PubMed]
- Jones ME, Schoemaker MJ, Wright LB, et al. Smoking and risk of breast cancer in the Generations Study cohort. Breast Cancer Res 2017;19:118. [Crossref] [PubMed]
- Dandamudi A, Tommie J, Nommsen-Rivers L, et al. Dietary Patterns and Breast Cancer Risk: A Systematic Review. Anticancer Res 2018;38:3209-22. [Crossref] [PubMed]
- Shan W, Ning C, Luo X, et al. Hyperinsulinemia is associated with endometrial hyperplasia and disordered proliferative endometrium: a prospective cross-sectional study. Gynecol Oncol 2014;132:606-10. [Crossref] [PubMed]
- Li X, Fan Y, Wang J, et al. Insulin Resistance and Metabolic Syndrome Increase the Risk of Relapse For Fertility Preserving Treatment in Atypical Endometrial Hyperplasia and Early Endometrial Cancer Patients. Front Oncol 2021;11:744689. [Crossref] [PubMed]
- Lu KH, Broaddus RR. Endometrial Cancer. N Engl J Med 2020;383:2053-64. [Crossref] [PubMed]
- Fortner RT, Sisti J, Chai B, et al. Parity, breastfeeding, and breast cancer risk by hormone receptor status and molecular phenotype: results from the Nurses’ Health Studies. Breast Cancer Res 2019;21:40. [Crossref] [PubMed]
- Crous-Bou M, Du M, Gunter MJ, et al. Coffee consumption and risk of endometrial cancer: a pooled analysis of individual participant data in the Epidemiology of Endometrial Cancer Consortium (E2C2). Am J Clin Nutr 2022;116:1219-28. [Crossref] [PubMed]
- Reid BM, Permuth JB, Sellers TA. Epidemiology of ovarian cancer: a review. Cancer Biol Med 2017;14:9-32. [Crossref] [PubMed]
- Nazari SS, Mukherjee P. An overview of mammographic density and its association with breast cancer. Breast Cancer 2018;25:259-67. [Crossref] [PubMed]
- Ye F, Liang Y, Wang Y, et al. Cancer-associated fibroblasts facilitate breast cancer progression through exosomal circTBPL1-mediated intercellular communication. Cell Death Dis 2023;14:471. [Crossref] [PubMed]
- Guo YP, Martin LJ, Hanna W, et al. Growth factors and stromal matrix proteins associated with mammographic densities. Cancer Epidemiol Biomarkers Prev 2001;10:243-8. [PubMed]
- Coradini D, Ambrogi F. Differential Expression of the Genes Coding for Adipokines and Epithelial Cell Polarity Components in Women With Low and High Mammographic Density. Clin Breast Cancer 2022;22:715-23. [Crossref] [PubMed]
- Lokate M, Peeters PH, Peelen LM, et al. Mammographic density and breast cancer risk: the role of the fat surrounding the fibroglandular tissue. Breast Cancer Res 2011;13:R103. [Crossref] [PubMed]
- Pettersson A, Hankinson SE, Willett WC, et al. Nondense mammographic area and risk of breast cancer. Breast Cancer Res 2011;13:R100. [Crossref] [PubMed]
- Giovannucci E, Harlan DM, Archer MC, et al. Diabetes and cancer: a consensus report. CA Cancer J Clin 2010;60:207-21. [Crossref] [PubMed]
- Wang T, Fahrmann JF, Lee H, et al. JAK/STAT3-Regulated Fatty Acid β-Oxidation Is Critical for Breast Cancer Stem Cell Self-Renewal and Chemoresistance. Cell Metab 2018;27:1357. [Crossref] [PubMed]
- Raut PK, Park PH. Globular adiponectin antagonizes leptin-induced growth of cancer cells by modulating inflammasomes activation: Critical role of HO-1 signaling. Biochem Pharmacol 2020;180:114186. [Crossref] [PubMed]
- Dąbruś D, Kiełbasiński R, Grabarek BO, et al. Evaluation of the Impact of Cisplatin on Variances in the Expression Pattern of Leptin-Related Genes in Endometrial Cancer Cells. Int J Mol Sci 2020;21:4135. [Crossref] [PubMed]
- Li R, Dong F, Zhang L, et al. Role of adipocytokines in endometrial cancer progression. Front Pharmacol 2022;13:1090227. [Crossref] [PubMed]
- Tsankof A, Tziomalos K. Adiponectin: A player in the pathogenesis of hormone-dependent cancers. Front Endocrinol (Lausanne) 2022;13:1018515. [Crossref] [PubMed]
- Dincer F, Atmaca H, Akman L, et al. Effects of leptin on the viability of human ovarian cancer cells and changes in cytokine expression levels. PeerJ 2023;11:e15246. [Crossref] [PubMed]
- Otokozawa S, Tanaka R, Akasaka H, et al. Associations of Serum Isoflavone, Adiponectin and Insulin Levels with Risk for Epithelial Ovarian Cancer: Results of a Case-control Study. Asian Pac J Cancer Prev 2015;16:4987-91. [Crossref] [PubMed]
- Wei R, Wong JPC, Kwok HF. Osteopontin -- a promising biomarker for cancer therapy. J Cancer 2017;8:2173-83. [Crossref] [PubMed]
- Rani S, Sehgal A, Kaur J, et al. Osteopontin as a Tumor Marker in Ovarian Cancer. J Midlife Health 2022;13:200-5. [Crossref] [PubMed]
- Cho S, Ahn YS, Choi YS, et al. Endometrial osteopontin mRNA expression and plasma osteopontin levels are increased in patients with endometriosis. Am J Reprod Immunol 2009;61:286-93. [Crossref] [PubMed]
- Gogola-Mruk J, Tworzydło W, Krawczyk K, et al. Visfatin induces ovarian cancer resistance to anoikis by regulating mitochondrial activity. Endocrine 2023;80:448-58. [Crossref] [PubMed]
- Ghaneialvar H, Shiri S, Kenarkoohi A, et al. Comparison of visfatin levels in patients with breast cancer and endometrial cancer with healthy individuals: A systematic review and meta-analysis. Health Sci Rep 2022;5:e895. [Crossref] [PubMed]
- Gourgue F, Mignion L, Van Hul M, et al. Obesity and triple-negative-breast-cancer: Is apelin a new key target? J Cell Mol Med 2020;24:10233-44. [Crossref] [PubMed]
- Grinstead C, Yoon S. Apelin, a Circulating Biomarker in Cancer Evaluation: A Systematic Review. Cancers (Basel) 2022;14:4656. [Crossref] [PubMed]
- Dogra S, Neelakantan D, Patel MM, et al. Adipokine Apelin/APJ Pathway Promotes Peritoneal Dissemination of Ovarian Cancer Cells by Regulating Lipid Metabolism. Mol Cancer Res 2021;19:1534-45. [Crossref] [PubMed]
- Altinkaya SO, Nergiz S, Küçük M, et al. Apelin levels are higher in obese patients with endometrial cancer. J Obstet Gynaecol Res 2015;41:294-300. [Crossref] [PubMed]
- Roy R, Yang J, Shimura T, et al. Escape from breast tumor dormancy: The convergence of obesity and menopause. Proc Natl Acad Sci U S A 2022;119:e2204758119. [Crossref] [PubMed]
- Wei CT, Tsai IT, Wu CC, et al. Elevated plasma level of neutrophil gelatinase-associated lipocalin (NGAL) in patients with breast cancer. Int J Med Sci 2021;18:2689-96. [Crossref] [PubMed]
- Yang J, Bielenberg DR, Rodig SJ, et al. Lipocalin 2 promotes breast cancer progression. Proc Natl Acad Sci U S A 2009;106:3913-8. [Crossref] [PubMed]
- Cymbaluk-Płoska A, Chudecka-Głaz A, Pius-Sadowska E, et al. The role of lipocalin-2 serum levels in the diagnostics of endometrial cancer. Cancer Biomark 2019;24:315-24. [Crossref] [PubMed]
- Hao P, Li H, Wu A, et al. Lipocalin2 promotes cell proliferation and migration in ovarian cancer through activation of the ERK/GSK3β/β-catenin signaling pathway. Life Sci 2020;262:118492. [Crossref] [PubMed]
- Hlavna M, Kohut L, Lipkova J, et al. Relationship of resistin levels with endometrial cancer risk. Neoplasma 2011;58:124-8. [Crossref] [PubMed]
- Chen Y, Xu J, Pan W, et al. Galectin-3 enhances trastuzumab resistance by regulating cancer malignancy and stemness in HER2-positive breast cancer cells. Thorac Cancer 2022;13:1961-73. [Crossref] [PubMed]
- Masoodi M, Shah ZA, Beigh AH, et al. Galectin-1 as a predictive biomarker in ovarian cancer. J Ovarian Res 2021;14:123. [Crossref] [PubMed]
- Cao XH, Chen X, Yang K, et al. Vaspin accelerates the proliferation, invasion and metastasis of Triple-Negative breast cancer through MiR-33a-5p/ABHD2. Cancer Med 2023;12:4530-42. [Crossref] [PubMed]
- Kozłowski M, Pietrzyk D, Rychlicka M, et al. Evaluation of Tissue Expression of Vaspin and Serum Vaspin Concentration as a Prognostic and Risk Factor in Endometrial Cancer. Cells 2022;11:3196. [Crossref] [PubMed]
- Kurowska P, Mlyczyńska E, Dawid M, et al. Role of vaspin in porcine ovary: effect on signaling pathways and steroid synthesis via GRP78 receptor and protein kinase A†. Biol Reprod 2020;102:1290-305. [Crossref] [PubMed]
- Bongrani A, Mellouk N, Ramé C, et al. Vaspin, a novel adipokine in woman granulosa cells physiology and PCOS pathogenesis? J Endocrinol 2021;249:57-70. [Crossref] [PubMed]
- Barron CC, Bilan PJ, Tsakiridis T, et al. Facilitative glucose transporters: Implications for cancer detection, prognosis and treatment. Metabolism 2016;65:124-39. [Crossref] [PubMed]
- Brown RS, Wahl RL. Overexpression of Glut-1 glucose transporter in human breast cancer. An immunohistochemical study. Cancer 1993;72:2979-85. [Crossref] [PubMed]
- Younes M, Brown RW, Mody DR, et al. GLUT1 expression in human breast carcinoma: correlation with known prognostic markers. Anticancer Res 1995;15:2895-8. [PubMed]
- Godoy A, Ulloa V, Rodríguez F, et al. Differential subcellular distribution of glucose transporters GLUT1-6 and GLUT9 in human cancer: ultrastructural localization of GLUT1 and GLUT5 in breast tumor tissues. J Cell Physiol 2006;207:614-27. [Crossref] [PubMed]
- Garrido P, Osorio FG, Morán J, et al. Loss of GLUT4 induces metabolic reprogramming and impairs viability of breast cancer cells. J Cell Physiol 2015;230:191-8. [Crossref] [PubMed]
- Shin E, Koo JS. Glucose Metabolism and Glucose Transporters in Breast Cancer. Front Cell Dev Biol 2021;9:728759. [Crossref] [PubMed]
- Lin Y, Lv F, Liu F, et al. High Expression of Pyruvate Kinase M2 is Associated with Chemosensitivity to Epirubicin and 5-Fluorouracil in Breast Cancer. J Cancer 2015;6:1130-9. [Crossref] [PubMed]
- Guan M, Tong Y, Guan M, et al. Lapatinib Inhibits Breast Cancer Cell Proliferation by Influencing PKM2 Expression. Technol Cancer Res Treat 2018;17:1533034617749418. [Crossref] [PubMed]
- Ying M, You D, Zhu X, et al. Lactate and glutamine support NADPH generation in cancer cells under glucose deprived conditions. Redox Biol 2021;46:102065. [Crossref] [PubMed]
- Becker LM, O’Connell JT, Vo AP, et al. Epigenetic Reprogramming of Cancer-Associated Fibroblasts Deregulates Glucose Metabolism and Facilitates Progression of Breast Cancer. Cell Rep 2020;31:107701. [Crossref] [PubMed]
- Zapf J, Schmid C, Froesch ER. Biological and immunological properties of insulin-like growth factors (IGF) I and II. Clin Endocrinol Metab 1984;13:3-30. [Crossref] [PubMed]
- Biello F, Platini F, D’Avanzo F, et al. Insulin/IGF Axis in Breast Cancer: Clinical Evidence and Translational Insights. Biomolecules 2021;11:125. [Crossref] [PubMed]
- Gennari A, Foca F, Zamarchi R, et al. Insulin-like growth factor-1 receptor (IGF-1R) expression on circulating tumor cells (CTCs) and metastatic breast cancer outcome: results from the TransMYME trial. Breast Cancer Res Treat 2020;181:61-8. [Crossref] [PubMed]
- Bowers LW, Rossi EL, O’Flanagan CH, et al. The Role of the Insulin/IGF System in Cancer: Lessons Learned from Clinical Trials and the Energy Balance-Cancer Link. Front Endocrinol (Lausanne) 2015;6:77. [Crossref] [PubMed]
- Ferguson RD, Novosyadlyy R, Fierz Y, et al. Hyperinsulinemia enhances c-Myc-mediated mammary tumor development and advances metastatic progression to the lung in a mouse model of type 2 diabetes. Breast Cancer Res 2012;14:R8. [Crossref] [PubMed]
- Zelenko Z, Gallagher EJ, Antoniou IM, et al. EMT reversal in human cancer cells after IR knockdown in hyperinsulinemic mice. Endocr Relat Cancer 2016;23:747-58. [Crossref] [PubMed]
- Varkaris A, Pazolli E, Gunaydin H, et al. Discovery and Clinical Proof-of-Concept of RLY-2608, a First-in-Class Mutant-Selective Allosteric PI3Kα Inhibitor That Decouples Antitumor Activity from Hyperinsulinemia. Cancer Discov 2024;14:240-57. [Crossref] [PubMed]
- Zhang T, Zhu X, Wu H, et al. Targeting the ROS/PI3K/AKT/HIF-1α/HK2 axis of breast cancer cells: Combined administration of Polydatin and 2-Deoxy-d-glucose. J Cell Mol Med 2019;23:3711-23. [Crossref] [PubMed]
- Lee J, Yesilkanal AE, Wynne JP, et al. Effective breast cancer combination therapy targeting BACH1 and mitochondrial metabolism. Nature 2019;568:254-8. [Crossref] [PubMed]
- Eiriksson FF, Nøhr MK, Costa M, et al. Lipidomic study of cell lines reveals differences between breast cancer subtypes. PLoS One 2020;15:e0231289. [Crossref] [PubMed]
- Baumann J, Kokabee M, Wong J, et al. Global metabolite profiling analysis of lipotoxicity in HER2/neu-positive breast cancer cells. Oncotarget 2018;9:27133-50. [Crossref] [PubMed]
- Yoon S, Lee MY, Park SW, et al. Up-regulation of acetyl-CoA carboxylase alpha and fatty acid synthase by human epidermal growth factor receptor 2 at the translational level in breast cancer cells. J Biol Chem 2007;282:26122-31. [Crossref] [PubMed]
- Alwarawrah Y, Hughes P, Loiselle D, et al. Fasnall, a Selective FASN Inhibitor, Shows Potent Anti-tumor Activity in the MMTV-Neu Model of HER2(+) Breast Cancer. Cell Chem Biol 2016;23:678-88. [Crossref] [PubMed]
- Lope V, Guerrero-Zotano Á, Casas A, et al. Serum Phospholipids Fatty Acids and Breast Cancer Risk by Pathological Subtype. Nutrients 2020;12:3132. [Crossref] [PubMed]
- Li L, Zheng X, Zhou Q, et al. Metabolomics-Based Discovery of Molecular Signatures for Triple Negative Breast Cancer in Asian Female Population. Sci Rep 2020;10:370. [Crossref] [PubMed]
- Hosokawa Y, Masaki N, Takei S, et al. Recurrent triple-negative breast cancer (TNBC) tissues contain a higher amount of phosphatidylcholine (32:1) than non-recurrent TNBC tissues. PLoS One 2017;12:e0183724. [Crossref] [PubMed]
- Ramadan S, Arm J, Silcock J, et al. Lipid and Metabolite Deregulation in the Breast Tissue of Women Carrying BRCA1 and BRCA2 Genetic Mutations. Radiology 2015;275:675-82. [Crossref] [PubMed]
- Anagnostou E, Miliaras D, Meditskou S, et al. Immunohistochemical investigation of metabolic markers fatty acid synthase (FASN) and glucose transporter 1 (GLUT1) in normal endometrium, endometrial hyperplasia, and endometrial malignancy. Hippokratia 2017;21:169-74. [PubMed]
- Frolova AI, Moley KH. Glucose transporters in the uterus: an analysis of tissue distribution and proposed physiological roles. Reproduction 2011;142:211-20. [Crossref] [PubMed]
- Cantuaria G, Fagotti A, Ferrandina G, et al. GLUT-1 expression in ovarian carcinoma: association with survival and response to chemotherapy. Cancer 2001;92:1144-50. [Crossref] [PubMed]
- Han J, Zhang L, Guo H, et al. Glucose promotes cell proliferation, glucose uptake and invasion in endometrial cancer cells via AMPK/mTOR/S6 and MAPK signaling. Gynecol Oncol 2015;138:668-75. [Crossref] [PubMed]
- Gu CJ, Xie F, Zhang B, et al. High Glucose Promotes Epithelial-Mesenchymal Transition of Uterus Endometrial Cancer Cells by Increasing ER/GLUT4-Mediated VEGF Secretion. Cell Physiol Biochem 2018;50:706-20. [Crossref] [PubMed]
- Al-Sharaky DR, Abdou AG, Wahed MM, et al. HIF-1α and GLUT-1 Expression in Atypical Endometrial Hyperplasia, Type I and II Endometrial Carcinoma: A Potential Role in Pathogenesis. J Clin Diagn Res 2016;10:EC20-7. [PubMed]
- Wahl H, Daudi S, Kshirsagar M, et al. Expression of metabolically targeted biomarkers in endometrial carcinoma. Gynecol Oncol 2010;116:21-7. [Crossref] [PubMed]
- Zhou K, Lin J, Dai M, et al. KIFC1 promotes aerobic glycolysis in endometrial cancer cells by regulating the c-myc pathway. J Bioenerg Biomembr 2021;53:703-13. [Crossref] [PubMed]
- Ben Ali F, Qmichou Z, Oukabli M, et al. Alteration of glucose metabolism and expression of glucose transporters in ovarian cancer. Explor Target Antitumor Ther 2024;5:384-99. [Crossref] [PubMed]
- Tsukioka M, Matsumoto Y, Noriyuki M, et al. Expression of glucose transporters in epithelial ovarian carcinoma: correlation with clinical characteristics and tumor angiogenesis. Oncol Rep 2007;18:361-7. [Crossref] [PubMed]
- Cantuaria G, Magalhaes A, Penalver M, et al. Expression of GLUT-1 glucose transporter in borderline and malignant epithelial tumors of the ovary. Gynecol Oncol 2000;79:33-7. [Crossref] [PubMed]
- Rudlowski C, Moser M, Becker AJ, et al. GLUT1 mRNA and protein expression in ovarian borderline tumors and cancer. Oncology 2004;66:404-10. [Crossref] [PubMed]
- van der Groep P, Bouter A, Menko FH, et al. High frequency of HIF-1alpha overexpression in BRCA1 related breast cancer. Breast Cancer Res Treat 2008;111:475-80. [Crossref] [PubMed]
- Pinciroli P, Alberti C, Sensi M, et al. An IL6-correlated signature in serous epithelial ovarian cancer associates with growth factor response. BMC Genomics 2013;14:508. [Crossref] [PubMed]
- Yu C, Niu X, Du Y, et al. IL-17A promotes fatty acid uptake through the IL-17A/IL-17RA/p-STAT3/FABP4 axis to fuel ovarian cancer growth in an adipocyte-rich microenvironment. Cancer Immunol Immunother 2020;69:115-26. [Crossref] [PubMed]
- Dupont J, Reverchon M, Cloix L, et al. Involvement of adipokines, AMPK, PI3K and the PPAR signaling pathways in ovarian follicle development and cancer. Int J Dev Biol 2012;56:959-67. [Crossref] [PubMed]
- Schumann T, Adhikary T, Wortmann A, et al. Deregulation of PPARβ/δ target genes in tumor-associated macrophages by fatty acid ligands in the ovarian cancer microenvironment. Oncotarget 2015;6:13416-33. [Crossref] [PubMed]
- Miranda F, Mannion D, Liu S, et al. Salt-Inducible Kinase 2 Couples Ovarian Cancer Cell Metabolism with Survival at the Adipocyte-Rich Metastatic Niche. Cancer Cell 2016;30:273-89. [Crossref] [PubMed]
- Tomek K, Wagner R, Varga F, et al. Blockade of fatty acid synthase induces ubiquitination and degradation of phosphoinositide-3-kinase signaling proteins in ovarian cancer. Mol Cancer Res 2011;9:1767-79. [Crossref] [PubMed]
- Bauerschlag DO, Maass N, Leonhardt P, et al. Fatty acid synthase overexpression: target for therapy and reversal of chemoresistance in ovarian cancer. J Transl Med 2015;13:146. [Crossref] [PubMed]
- Jiang L, Fang X, Wang H, et al. Ovarian Cancer-Intrinsic Fatty Acid Synthase Prevents Anti-tumor Immunity by Disrupting Tumor-Infiltrating Dendritic Cells. Front Immunol 2018;9:2927. [Crossref] [PubMed]
- Papaevangelou E, Almeida GS, Box C, et al. The effect of FASN inhibition on the growth and metabolism of a cisplatin-resistant ovarian carcinoma model. Int J Cancer 2018;143:992-1002. [Crossref] [PubMed]
- Tan Y, Li J, Zhao G, et al. Metabolic reprogramming from glycolysis to fatty acid uptake and beta-oxidation in platinum-resistant cancer cells. Nat Commun 2022;13:4554. [Crossref] [PubMed]
Cite this article as: Rodríguez YM, Koomson AA, Perry RJ. Breast cancer shares many epidemiological, lifestyle, and local hormonal and metabolic underpinnings with endometrial and ovarian cancer: a narrative review. Transl Breast Cancer Res 2025;6:8.